Three distinct mechanisms generate oxygen free radicals in neurons and contribute to cell death during anoxia and reoxygenation
- PMID: 17267568
- PMCID: PMC6673180
- DOI: 10.1523/JNEUROSCI.4468-06.2007
Three distinct mechanisms generate oxygen free radicals in neurons and contribute to cell death during anoxia and reoxygenation
Abstract
Ischemia is a major cause of brain damage, and patient management is complicated by the paradoxical injury that results from reoxygenation. We have now explored the generation of reactive oxygen species (ROS) in hippocampal and cortical neurons in culture in response to oxygen and glucose deprivation or metabolic inhibition and reoxygenation. Fluorescence microscopy was used to measure the rate of ROS generation using hydroethidine, dicarboxyfluorescein diacetate, or MitoSOX. ROS generation was correlated with changing mitochondrial potential (rhodamine 123), [Ca2+]c (fluo-4, fura-2, or Indo-1), or ATP consumption, indicated by increased [Mg2+]c. We found that three distinct mechanisms contribute to neuronal injury by generating ROS and oxidative stress, each operating at a different stage of ischemia and reperfusion. In response to hypoxia, mitochondria generate an initial burst of ROS, which is curtailed once mitochondria depolarize or prevented by previous depolarization with uncoupler. A second phase of ROS generation that followed after a delay was blocked by the xanthine oxidase (XO) inhibitor oxypurinol. This phase correlated with a rise in [Mg2+]c, suggesting XO activation by accumulating products of ATP consumption. A third phase of ROS generation appeared at reoxygenation. This was blocked by NADPH oxidase inhibitors and was absent in cells from gp91(phox-/-) knock-out mice. It was Ca2+ dependent, suggesting activation by increased [Ca2+]c during anoxia, itself partly attributable to glutamate release. Inhibition of either the NADPH oxidase or XO was significantly neuroprotective. Thus, oxidative stress contributes to cell death over and above the injury attributable to energy deprivation.
Figures
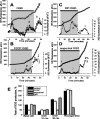
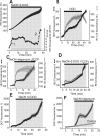
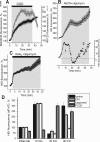
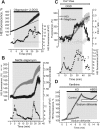
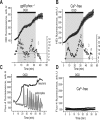
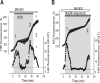
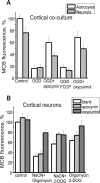
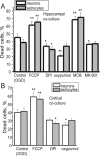
Similar articles
-
Neuroprotective effects of gallic acid against hypoxia/reoxygenation-induced mitochondrial dysfunctions in vitro and cerebral ischemia/reperfusion injury in vivo.Brain Res. 2014 Nov 17;1589:126-39. doi: 10.1016/j.brainres.2014.09.039. Epub 2014 Sep 22. Brain Res. 2014. PMID: 25251593
-
Reoxygenation after hypoxia and glucose depletion causes reactive oxygen species production by mitochondria in HUVEC.Am J Physiol Regul Integr Comp Physiol. 2004 Nov;287(5):R1037-43. doi: 10.1152/ajpregu.00048.2004. Epub 2004 Jun 17. Am J Physiol Regul Integr Comp Physiol. 2004. PMID: 15205181
-
Allopurinol modulates reactive oxygen species generation and Ca2+ overload in ischemia-reperfused heart and hypoxia-reoxygenated cardiomyocytes.Eur J Pharmacol. 2006 Mar 27;535(1-3):212-9. doi: 10.1016/j.ejphar.2006.01.013. Epub 2006 Mar 6. Eur J Pharmacol. 2006. PMID: 16516885
-
Reactive species mechanisms of cellular hypoxia-reoxygenation injury.Am J Physiol Cell Physiol. 2002 Feb;282(2):C227-41. doi: 10.1152/ajpcell.00112.2001. Am J Physiol Cell Physiol. 2002. PMID: 11788333 Review.
-
Reperfusion injury and reactive oxygen species: The evolution of a concept.Redox Biol. 2015 Dec;6:524-551. doi: 10.1016/j.redox.2015.08.020. Epub 2015 Oct 8. Redox Biol. 2015. PMID: 26484802 Free PMC article. Review.
Cited by
-
Chronic renal ischemia in humans: can cell therapy repair the kidney in occlusive renovascular disease?Physiology (Bethesda). 2015 May;30(3):175-82. doi: 10.1152/physiol.00065.2013. Physiology (Bethesda). 2015. PMID: 25933818 Free PMC article. Review.
-
Specific inhibition of NADPH oxidase 2 modifies chronic epilepsy.Redox Biol. 2022 Dec;58:102549. doi: 10.1016/j.redox.2022.102549. Epub 2022 Nov 28. Redox Biol. 2022. PMID: 36459714 Free PMC article.
-
Brain Energy Deficit as a Source of Oxidative Stress in Migraine: A Molecular Basis for Migraine Susceptibility.Neurochem Res. 2021 Aug;46(8):1913-1932. doi: 10.1007/s11064-021-03335-9. Epub 2021 Apr 30. Neurochem Res. 2021. PMID: 33939061 Review.
-
Astrocyte metabolism and signaling during brain ischemia.Nat Neurosci. 2007 Nov;10(11):1377-86. doi: 10.1038/nn2004. Nat Neurosci. 2007. PMID: 17965658 Free PMC article. Review.
-
Reversible cyclosporin A-sensitive mitochondrial depolarization occurs within minutes of stroke onset in mouse somatosensory cortex in vivo: a two-photon imaging study.J Biol Chem. 2009 Dec 25;284(52):36109-36117. doi: 10.1074/jbc.M109.055301. Epub 2009 Nov 5. J Biol Chem. 2009. PMID: 19892710 Free PMC article.
References
Publication types
MeSH terms
Substances
Grants and funding
LinkOut - more resources
Full Text Sources
Other Literature Sources
Research Materials
Miscellaneous