Molecular bases of viral RNA targeting by viral small interfering RNA-programmed RISC
- PMID: 17267504
- PMCID: PMC1866121
- DOI: 10.1128/JVI.02383-06
Molecular bases of viral RNA targeting by viral small interfering RNA-programmed RISC
Abstract
RNA silencing is conserved in a broad range of eukaryotes and operates in the development and maintenance of genome integrity in many organisms. Plants have adapted this system for antiviral defense, and plant viruses have in turn developed mechanisms to suppress RNA silencing. RNA silencing-related RNA inactivation is likely based on target RNA cleavage or translational arrest. Although it is widely assumed that virus-induced gene silencing (VIGS) promotes the endonucleolytic cleavage of the viral RNA genome, this popular assumption has never been tested experimentally. Here we analyzed the viral RNA targeting by VIGS in tombusvirus-infected plants, and we show evidence that antiviral response of VIGS is based on viral RNA cleavage by RNA-induced silencing effector complex (RISC) programmed by virus-specific small interfering RNAs (siRNAs). In addition, we found that the RISC-mediated cleavages do not occur randomly on the viral genome. Indeed, sequence analysis of cloned cleavage products identified hot spots for target RNA cleavage, and the regions of specific RISC-mediated cleavages are asymmetrically distributed along the positive- and negative-sense viral RNA strands. In addition, we identified viral siRNAs containing high-molecular-mass protein complexes purified from the recovery leaves of the silencing suppressor mutant virus-infected plants. Strikingly, these large nucleoproteins cofractionated with microRNA-containing complexes, suggesting that these nucleoproteins are silencing related effector complexes.
Figures
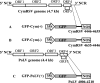
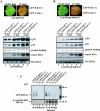
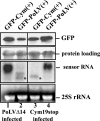
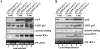
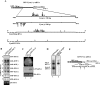
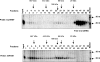
Similar articles
-
Plant virus-derived small interfering RNAs originate predominantly from highly structured single-stranded viral RNAs.J Virol. 2005 Jun;79(12):7812-8. doi: 10.1128/JVI.79.12.7812-7818.2005. J Virol. 2005. PMID: 15919934 Free PMC article.
-
AGO/RISC-mediated antiviral RNA silencing in a plant in vitro system.Nucleic Acids Res. 2013 May;41(9):5090-103. doi: 10.1093/nar/gkt193. Epub 2013 Mar 27. Nucleic Acids Res. 2013. PMID: 23535144 Free PMC article.
-
Molecular mechanism of RNA silencing suppression mediated by p19 protein of tombusviruses.EMBO J. 2004 Feb 25;23(4):876-84. doi: 10.1038/sj.emboj.7600096. Epub 2004 Feb 19. EMBO J. 2004. PMID: 14976549 Free PMC article.
-
Effects and side-effects of viral RNA silencing suppressors on short RNAs.Trends Plant Sci. 2004 Feb;9(2):76-83. doi: 10.1016/j.tplants.2003.12.010. Trends Plant Sci. 2004. PMID: 15102373 Review.
-
RNAi and retroviruses: are they in RISC?Biomol Concepts. 2013 Feb;4(1):43-52. doi: 10.1515/bmc-2012-0041. Biomol Concepts. 2013. PMID: 25436564 Review.
Cited by
-
Polycistronic artificial miRNA-mediated resistance to Wheat dwarf virus in barley is highly efficient at low temperature.Mol Plant Pathol. 2016 Apr;17(3):427-37. doi: 10.1111/mpp.12291. Epub 2015 Jul 30. Mol Plant Pathol. 2016. PMID: 26136043 Free PMC article.
-
Characterization of hypovirus-derived small RNAs generated in the chestnut blight fungus by an inducible DCL-2-dependent pathway.J Virol. 2008 Mar;82(6):2613-9. doi: 10.1128/JVI.02324-07. Epub 2008 Jan 16. J Virol. 2008. PMID: 18199652 Free PMC article.
-
RNA-based viral immunity initiated by the Dicer family of host immune receptors.Immunol Rev. 2009 Jan;227(1):176-88. doi: 10.1111/j.1600-065X.2008.00722.x. Immunol Rev. 2009. PMID: 19120484 Free PMC article. Review.
-
RNA silencing suppression by plant pathogens: defence, counter-defence and counter-counter-defence.Nat Rev Microbiol. 2013 Nov;11(11):745-60. doi: 10.1038/nrmicro3120. Nat Rev Microbiol. 2013. PMID: 24129510 Review.
-
Small-RNA deep sequencing reveals Arctium tomentosum as a natural host of Alstroemeria virus X and a new putative Emaravirus.PLoS One. 2012;7(8):e42758. doi: 10.1371/journal.pone.0042758. Epub 2012 Aug 17. PLoS One. 2012. PMID: 22912734 Free PMC article.
References
Publication types
MeSH terms
Substances
LinkOut - more resources
Full Text Sources