Identification of the molecular switch that regulates access of 5alpha-DHT to the androgen receptor
- PMID: 17223255
- PMCID: PMC1857325
- DOI: 10.1016/j.mce.2006.12.007
Identification of the molecular switch that regulates access of 5alpha-DHT to the androgen receptor
Abstract
Pairs of hydroxysteroid dehydrogenases (HSDs) govern ligand access to steroid receptors in target tissues and act as molecular switches. By acting as reductases or oxidases, HSDs convert potent ligands into their cognate inactive metabolites or vice versa. This pre-receptor regulation of steroid hormone action may have profound effects on hormonal response. We have identified the HSDs responsible for regulating ligand access to the androgen receptor (AR) in human prostate. Type 3 3alpha-hydroxysteroid dehydrogenase (aldo-keto reductase 1C2) acts solely as a reductase to convert 5alpha-dihydrotestosterone (DHT), a potent ligand for the AR (K(d)=10(-11)M for the AR), to the inactive androgen 3alpha-androstanediol (K(d)=10(-6)M for the AR); while RoDH like 3alpha-HSD (a short-chain dehydrogenase/reductase (SDR)) acts solely as an oxidase to convert 3alpha-androstanediol back to 5alpha-DHT. Our studies suggest that aldo-keto reductase (AKRs) and SDRs function as reductases and oxidases, respectively, to control ligand access to nuclear receptors.
Figures
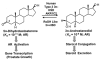
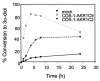
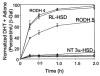
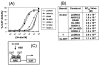
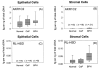
Similar articles
-
Human type 3 3alpha-hydroxysteroid dehydrogenase (aldo-keto reductase 1C2) and androgen metabolism in prostate cells.Endocrinology. 2003 Jul;144(7):2922-32. doi: 10.1210/en.2002-0032. Endocrinology. 2003. PMID: 12810547
-
Role of human type 3 3alpha-hydroxysteroid dehydrogenase (AKR1C2) in androgen metabolism of prostate cancer cells.Chem Biol Interact. 2003 Feb 1;143-144:401-9. doi: 10.1016/s0009-2797(02)00179-5. Chem Biol Interact. 2003. PMID: 12604227
-
Pre-receptor regulation of the androgen receptor.Mol Cell Endocrinol. 2008 Jan 16;281(1-2):1-8. doi: 10.1016/j.mce.2007.10.008. Epub 2007 Oct 22. Mol Cell Endocrinol. 2008. PMID: 18060684 Free PMC article. Review.
-
Identification of the major oxidative 3alpha-hydroxysteroid dehydrogenase in human prostate that converts 5alpha-androstane-3alpha,17beta-diol to 5alpha-dihydrotestosterone: a potential therapeutic target for androgen-dependent disease.Mol Endocrinol. 2006 Feb;20(2):444-58. doi: 10.1210/me.2005-0287. Epub 2005 Sep 22. Mol Endocrinol. 2006. PMID: 16179381
-
Steroid 5alpha-reductases and 3alpha-hydroxysteroid dehydrogenases: key enzymes in androgen metabolism.Best Pract Res Clin Endocrinol Metab. 2001 Mar;15(1):79-94. doi: 10.1053/beem.2001.0120. Best Pract Res Clin Endocrinol Metab. 2001. PMID: 11469812 Review.
Cited by
-
Hormonal gatekeeping via the blood-brain barrier governs caste-specific behavior in ants.Cell. 2023 Sep 28;186(20):4289-4309.e23. doi: 10.1016/j.cell.2023.08.002. Epub 2023 Sep 7. Cell. 2023. PMID: 37683635 Free PMC article.
-
The Potential Tumor-Suppressor DHRS7 Inversely Correlates with EGFR Expression in Prostate Cancer Cells and Tumor Samples.Cancers (Basel). 2022 Jun 23;14(13):3074. doi: 10.3390/cancers14133074. Cancers (Basel). 2022. PMID: 35804847 Free PMC article.
-
Intracrine androgen biosynthesis and drug resistance.Cancer Drug Resist. 2020 Nov 3;3(4):912-929. doi: 10.20517/cdr.2020.60. eCollection 2020. Cancer Drug Resist. 2020. PMID: 35582223 Free PMC article. Review.
-
Using biochemistry and biophysics to extinguish androgen receptor signaling in prostate cancer.J Biol Chem. 2021 Jan-Jun;296:100240. doi: 10.1074/jbc.REV120.012411. Epub 2021 Jan 9. J Biol Chem. 2021. PMID: 33384381 Free PMC article. Review.
-
Amide Bond Bioisosteres: Strategies, Synthesis, and Successes.J Med Chem. 2020 Nov 12;63(21):12290-12358. doi: 10.1021/acs.jmedchem.0c00530. Epub 2020 Aug 4. J Med Chem. 2020. PMID: 32686940 Free PMC article. Review.
References
-
- Agarwal AK, Auchus RJ. Minireview: Cellular redox state regulates hydroxysteroid dehydrogenase activity and intracellular hormone potency. Endocrinology. 2005;146:2531–2538. - PubMed
-
- Bauman DR, Steckelbroeck S, Williams MV, Peehl DM, Penning TM. Identification of the major oxidative 3α-hydroxysteroid dehydrogenase in human prostate that converts 5α-androstane-3α,17β-diol to 5α-dihydrotestosterone: A potential therapeutic target for androgen dependent disease. Mol Endocrinol. 2006a;20:444–458. - PubMed
-
- Buaman DP, Steckelbroeck S, Peehl DM, Penning TM. Transcript profiling of the androgen signal in normal prostate, benign prostatic hyperplasia, and prostate cancer. Endocrinology. 2006b Epub Ahead of Print September 7, 2006. - PubMed
-
- Biswas MG, Russell DW. Expression cloning and characterization of oxidative 17β– and 3α-hydroxysteroid dehydrogenases from rat and human prostate. J Biol Chem. 1997;272:15959–15966. - PubMed
Publication types
MeSH terms
Substances
Grants and funding
LinkOut - more resources
Full Text Sources
Research Materials
Miscellaneous