L13a blocks 48S assembly: role of a general initiation factor in mRNA-specific translational control
- PMID: 17218275
- PMCID: PMC1810376
- DOI: 10.1016/j.molcel.2006.11.028
L13a blocks 48S assembly: role of a general initiation factor in mRNA-specific translational control
Abstract
Transcript-specific translational control restricts macrophage inflammatory gene expression. The proinflammatory cytokine interferon-gamma induces phosphorylation of ribosomal protein L13a and translocation from the 60S ribosomal subunit to the interferon-gamma-activated inhibitor of translation (GAIT) complex. This complex binds the 3'UTR of ceruloplasmin mRNA and blocks its translation. Here, we elucidate the molecular mechanism underlying repression by L13a. Translation of the GAIT element-containing reporter mRNA is sensitive to L13a-mediated silencing when driven by internal ribosome entry sites (IRESs) that require initiation factor eIF4G, but is resistant to silencing when driven by eIF4F-independent IRESs, demonstrating a critical role for eIF4G. Interaction of L13a with eIF4G blocks 43S recruitment without suppressing eIF4F complex formation. eIF4G attack, e.g., by virus, stress, or caspases, is a well-known mechanism of global inhibition of protein synthesis. However, our studies reveal a unique mechanism in which targeting of eIF4G by mRNA-bound L13a elicits transcript-specific translational repression.
Figures
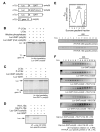
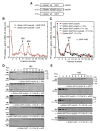
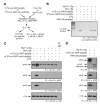
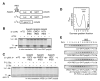
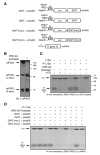
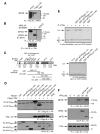
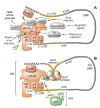
Similar articles
-
Eukaryotic translation initiation factor 3 (eIF3) and eIF2 can promote mRNA binding to 40S subunits independently of eIF4G in yeast.Mol Cell Biol. 2006 Feb;26(4):1355-72. doi: 10.1128/MCB.26.4.1355-1372.2006. Mol Cell Biol. 2006. PMID: 16449648 Free PMC article.
-
Regulated release of L13a from the 60S ribosomal subunit as a mechanism of transcript-specific translational control.Cell. 2003 Oct 17;115(2):187-98. doi: 10.1016/s0092-8674(03)00773-6. Cell. 2003. PMID: 14567916
-
Increase in cap- and IRES-dependent protein synthesis by overproduction of translation initiation factor eIF4G.Biochem Biophys Res Commun. 2000 Oct 14;277(1):117-23. doi: 10.1006/bbrc.2000.3637. Biochem Biophys Res Commun. 2000. PMID: 11027650
-
eIF4 initiation factors: effectors of mRNA recruitment to ribosomes and regulators of translation.Annu Rev Biochem. 1999;68:913-63. doi: 10.1146/annurev.biochem.68.1.913. Annu Rev Biochem. 1999. PMID: 10872469 Review.
-
eIF4G: a multipurpose ribosome adapter?Science. 1997 Jan 24;275(5299):500-1. doi: 10.1126/science.275.5299.500. Science. 1997. PMID: 9019810 Review. No abstract available.
Cited by
-
Specialized ribosomes: a new frontier in gene regulation and organismal biology.Nat Rev Mol Cell Biol. 2012 May 23;13(6):355-69. doi: 10.1038/nrm3359. Nat Rev Mol Cell Biol. 2012. PMID: 22617470 Free PMC article. Review.
-
From cis-regulatory elements to complex RNPs and back.Cold Spring Harb Perspect Biol. 2012 Jul 1;4(7):a012245. doi: 10.1101/cshperspect.a012245. Cold Spring Harb Perspect Biol. 2012. PMID: 22751153 Free PMC article.
-
Glycogen synthase kinase 3 promotes p53 mRNA translation via phosphorylation of RNPC1.Genes Dev. 2013 Oct 15;27(20):2246-58. doi: 10.1101/gad.221739.113. Genes Dev. 2013. PMID: 24142875 Free PMC article.
-
Regulation and deregulation of mRNA translation during myeloid maturation.Exp Hematol. 2011 Feb;39(2):133-41. doi: 10.1016/j.exphem.2010.10.011. Epub 2010 Nov 18. Exp Hematol. 2011. PMID: 21093533 Free PMC article. Review.
-
Genome-wide polysome profiling reveals an inflammation-responsive posttranscriptional operon in gamma interferon-activated monocytes.Mol Cell Biol. 2009 Jan;29(2):458-70. doi: 10.1128/MCB.00824-08. Epub 2008 Nov 10. Mol Cell Biol. 2009. PMID: 19001086 Free PMC article.
References
-
- Anthony DD, Merrick WC. Analysis of 40 S and 80 S complexes with mRNA as measured by sucrose density gradients and primer extension inhibition. J Biol Chem. 1992;267:1554–1562. - PubMed
-
- Beckmann K, Grskovic M, Gebauer F, Hentze MW. A dual inhibitory mechanism restricts msl-2 mRNA translation for dosage compensation in Drosophila. Cell. 2005;122:529–540. - PubMed
-
- Cho PF, Poulin F, Cho-Park YA, Cho-Park IB, Chicoine JD, Lasko P, Sonenberg N. A new paradigm for translational control: inhibition via 5′-3′ mRNA tethering by Bicoid and the eIF4E cognate 4EHP. Cell. 2005;121:411–423. - PubMed
Publication types
MeSH terms
Substances
Grants and funding
LinkOut - more resources
Full Text Sources
Other Literature Sources
Molecular Biology Databases