Structural insight into the ESCRT-I/-II link and its role in MVB trafficking
- PMID: 17215868
- PMCID: PMC1783442
- DOI: 10.1038/sj.emboj.7601501
Structural insight into the ESCRT-I/-II link and its role in MVB trafficking
Abstract
ESCRT (endosomal sorting complex required for transport) complexes orchestrate efficient sorting of ubiquitinated transmembrane receptors to lysosomes via multivesicular bodies (MVBs). Yeast ESCRT-I and ESCRT-II interact directly in vitro; however, this association is not detected in yeast cytosol. To gain understanding of the molecular mechanisms of this link, we have characterised the ESCRT-I/-II supercomplex and determined the crystal structure of its interface. The link is formed by the vacuolar protein sorting (Vps)28 C-terminus (ESCRT-I) binding with nanomolar affinity to the Vps36-NZF-N zinc-finger domain (ESCRT-II). A hydrophobic patch on the Vps28-CT four-helix bundle contacts the hydrophobic knuckles of Vps36-NZF-N. Mutation of the ESCRT-I/-II link results in a cargo-sorting defect in yeast. Interestingly, the two Vps36 NZF domains, NZF-N and NZF-C, despite having the same core fold, use distinct surfaces to bind ESCRT-I or ubiquitinated cargo. We also show that a new component of ESCRT-I, Mvb12 (YGR206W), engages ESCRT-I directly with nanomolar affinity to form a 1:1:1:1 heterotetramer. Mvb12 does not affect the affinity of ESCRT-I for ESCRT-II in vitro. Our data suggest a complex regulatory mechanism for the ESCRT-I/-II link in yeast.
Figures
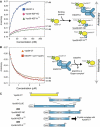
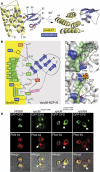
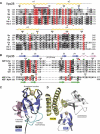
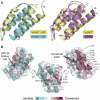
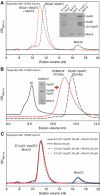
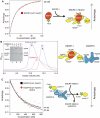
Similar articles
-
Efficient cargo sorting by ESCRT-I and the subsequent release of ESCRT-I from multivesicular bodies requires the subunit Mvb12.Mol Biol Cell. 2007 Feb;18(2):636-45. doi: 10.1091/mbc.e06-07-0588. Epub 2006 Nov 29. Mol Biol Cell. 2007. PMID: 17135292 Free PMC article.
-
ESCRT-I core and ESCRT-II GLUE domain structures reveal role for GLUE in linking to ESCRT-I and membranes.Cell. 2006 Apr 7;125(1):99-111. doi: 10.1016/j.cell.2006.01.047. Cell. 2006. PMID: 16615893
-
Vps27-Hse1 and ESCRT-I complexes cooperate to increase efficiency of sorting ubiquitinated proteins at the endosome.J Cell Biol. 2003 Oct 27;163(2):237-43. doi: 10.1083/jcb.200305007. J Cell Biol. 2003. PMID: 14581452 Free PMC article.
-
The emerging shape of the ESCRT machinery.Nat Rev Mol Cell Biol. 2007 May;8(5):355-68. doi: 10.1038/nrm2162. Nat Rev Mol Cell Biol. 2007. PMID: 17450176 Review.
-
Structural studies of phosphoinositide 3-kinase-dependent traffic to multivesicular bodies.Biochem Soc Symp. 2007;(74):47-57. doi: 10.1042/BSS0740047. Biochem Soc Symp. 2007. PMID: 17233579 Review.
Cited by
-
Why Cells and Viruses Cannot Survive without an ESCRT.Cells. 2021 Feb 24;10(3):483. doi: 10.3390/cells10030483. Cells. 2021. PMID: 33668191 Free PMC article. Review.
-
Exosomal transmission of viruses, a two-edged biological sword.Cell Commun Signal. 2023 Jan 23;21(1):19. doi: 10.1186/s12964-022-01037-5. Cell Commun Signal. 2023. PMID: 36691072 Free PMC article. Review.
-
Molecular architecture and functional model of the complete yeast ESCRT-I heterotetramer.Cell. 2007 May 4;129(3):485-98. doi: 10.1016/j.cell.2007.03.016. Epub 2007 Apr 19. Cell. 2007. PMID: 17442384 Free PMC article.
-
Component Interaction of ESCRT Complexes Is Essential for Endocytosis-Dependent Growth, Reproduction, DON Production and Full Virulence in Fusarium graminearum.Front Microbiol. 2019 Feb 12;10:180. doi: 10.3389/fmicb.2019.00180. eCollection 2019. Front Microbiol. 2019. PMID: 30809208 Free PMC article.
-
Cellular ESCRT components are recruited to regulate the endocytic trafficking and RNA replication compartment assembly during classical swine fever virus infection.PLoS Pathog. 2022 Feb 4;18(2):e1010294. doi: 10.1371/journal.ppat.1010294. eCollection 2022 Feb. PLoS Pathog. 2022. PMID: 35120190 Free PMC article.
References
-
- Adams SR, Campbell RE, Gross LA, Martin BR, Walkup GK, Yao Y, Llopis J, Tsien RY (2002) New biarsenical ligands and tetracysteine motifs for protein labeling in vitro and in vivo: synthesis and biological applications. J Am Chem Soc 124: 6063–6076 - PubMed
-
- Babst M, Katzmann DJ, Estepa-Sabal EJ, Meerloo T, Emr SD (2002a) ESCRT-III: an endosome-associated heterooligomeric protein complex required for MVB sorting. Dev Cell 3: 271–282 - PubMed
-
- Babst M, Katzmann DJ, Snyder WB, Wendland B, Emr SD (2002b) Endosome-associated complex, ESCRT-II, recruits transport machinery for protein sorting at the multivesicular body. Dev Cell 3: 283–289 - PubMed
Publication types
MeSH terms
Substances
Grants and funding
LinkOut - more resources
Full Text Sources
Other Literature Sources
Molecular Biology Databases