Vaccine efficacy in senescent mice challenged with recombinant SARS-CoV bearing epidemic and zoonotic spike variants
- PMID: 17194199
- PMCID: PMC1716185
- DOI: 10.1371/journal.pmed.0030525
Vaccine efficacy in senescent mice challenged with recombinant SARS-CoV bearing epidemic and zoonotic spike variants
Erratum in
- PLoS Med. 2007 Feb;4(2):e80
Abstract
Background: In 2003, severe acute respiratory syndrome coronavirus (SARS-CoV) was identified as the etiological agent of severe acute respiratory syndrome, a disease characterized by severe pneumonia that sometimes results in death. SARS-CoV is a zoonotic virus that crossed the species barrier, most likely originating from bats or from other species including civets, raccoon dogs, domestic cats, swine, and rodents. A SARS-CoV vaccine should confer long-term protection, especially in vulnerable senescent populations, against both the 2003 epidemic strains and zoonotic strains that may yet emerge from animal reservoirs. We report the comprehensive investigation of SARS vaccine efficacy in young and senescent mice following homologous and heterologous challenge.
Methods and findings: Using Venezuelan equine encephalitis virus replicon particles (VRP) expressing the 2003 epidemic Urbani SARS-CoV strain spike (S) glycoprotein (VRP-S) or the nucleocapsid (N) protein from the same strain (VRP-N), we demonstrate that VRP-S, but not VRP-N vaccines provide complete short- and long-term protection against homologous strain challenge in young and senescent mice. To test VRP vaccine efficacy against a heterologous SARS-CoV, we used phylogenetic analyses, synthetic biology, and reverse genetics to construct a chimeric virus (icGDO3-S) encoding a synthetic S glycoprotein gene of the most genetically divergent human strain, GDO3, which clusters among the zoonotic SARS-CoV. icGD03-S replicated efficiently in human airway epithelial cells and in the lungs of young and senescent mice, and was highly resistant to neutralization with antisera directed against the Urbani strain. Although VRP-S vaccines provided complete short-term protection against heterologous icGD03-S challenge in young mice, only limited protection was seen in vaccinated senescent animals. VRP-N vaccines not only failed to protect from homologous or heterologous challenge, but resulted in enhanced immunopathology with eosinophilic infiltrates within the lungs of SARS-CoV-challenged mice. VRP-N-induced pathology presented at day 4, peaked around day 7, and persisted through day 14, and was likely mediated by cellular immune responses.
Conclusions: This study identifies gaps and challenges in vaccine design for controlling future SARS-CoV zoonosis, especially in vulnerable elderly populations. The availability of a SARS-CoV virus bearing heterologous S glycoproteins provides a robust challenge inoculum for evaluating vaccine efficacy against zoonotic strains, the most likely source of future outbreaks.
Conflict of interest statement
Figures
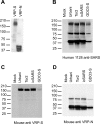
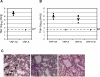
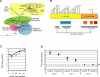
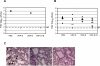
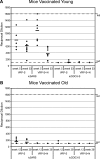
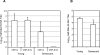
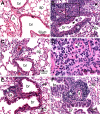
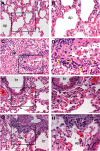
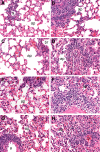
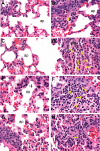
Similar articles
-
Successful vaccination strategies that protect aged mice from lethal challenge from influenza virus and heterologous severe acute respiratory syndrome coronavirus.J Virol. 2011 Jan;85(1):217-30. doi: 10.1128/JVI.01805-10. Epub 2010 Oct 27. J Virol. 2011. PMID: 20980507 Free PMC article.
-
Development of a Broadly Accessible Venezuelan Equine Encephalitis Virus Replicon Particle Vaccine Platform.J Virol. 2018 May 14;92(11):e00027-18. doi: 10.1128/JVI.00027-18. Print 2018 Jun 1. J Virol. 2018. PMID: 29540599 Free PMC article.
-
Synthetic reconstruction of zoonotic and early human severe acute respiratory syndrome coronavirus isolates that produce fatal disease in aged mice.J Virol. 2007 Jul;81(14):7410-23. doi: 10.1128/JVI.00505-07. Epub 2007 May 16. J Virol. 2007. PMID: 17507479 Free PMC article.
-
Severe acute respiratory syndrome vaccine development: experiences of vaccination against avian infectious bronchitis coronavirus.Avian Pathol. 2003 Dec;32(6):567-82. doi: 10.1080/03079450310001621198. Avian Pathol. 2003. PMID: 14676007 Free PMC article. Review.
-
Vaccine design for severe acute respiratory syndrome coronavirus.Viral Immunol. 2005;18(2):327-32. doi: 10.1089/vim.2005.18.327. Viral Immunol. 2005. PMID: 16035944 Review.
Cited by
-
Design and Application of Biosafe Coronavirus Engineering Systems without Virulence.Viruses. 2024 Apr 24;16(5):659. doi: 10.3390/v16050659. Viruses. 2024. PMID: 38793541 Free PMC article. Review.
-
Adjuvant-dependent impact of inactivated SARS-CoV-2 vaccines during heterologous infection by a SARS-related coronavirus.Nat Commun. 2024 May 3;15(1):3738. doi: 10.1038/s41467-024-47450-x. Nat Commun. 2024. PMID: 38702297 Free PMC article.
-
Characterization of humoral immune responses against SARS-CoV-2 accessory proteins in infected patients and mouse model.Virol Sin. 2024 Jun;39(3):414-421. doi: 10.1016/j.virs.2024.04.005. Epub 2024 Apr 26. Virol Sin. 2024. PMID: 38677713 Free PMC article.
-
Seroprevalence of anti-SARS-CoV-2 IgG antibodies pre- and post-COVID-19 vaccination in staff members of Bandar Abbas Children's Hospital.BMC Infect Dis. 2024 Feb 23;24(1):253. doi: 10.1186/s12879-023-08863-z. BMC Infect Dis. 2024. PMID: 38395759 Free PMC article.
-
Adjuvant-dependent effects on the safety and efficacy of inactivated SARS-CoV-2 vaccines during heterologous infection by a SARS-related coronavirus.Res Sq [Preprint]. 2023 Oct 27:rs.3.rs-3401539. doi: 10.21203/rs.3.rs-3401539/v1. Res Sq. 2023. Update in: Nat Commun. 2024 May 3;15(1):3738. doi: 10.1038/s41467-024-47450-x. PMID: 37961507 Free PMC article. Updated. Preprint.
References
-
- Drosten C, Gunther S, Preiser W, van der Werf S, Brodt HR, et al. Identification of a novel coronavirus in patients with severe acute respiratory syndrome. N Engl J Med. 2003;348:1967–1976. - PubMed
-
- Ksiazek TG, Erdman D, Goldsmith CS, Zaki SR, Peret T, et al. A novel coronavirus associated with severe acute respiratory syndrome. N Engl J Med. 2003;348:1953–1966. - PubMed
-
- Han Y, Geng H, Feng W, Tang X, Ou A, et al. A follow-up study of 69 discharged SARS patients. J Tradit Chin Med. 2003;23:214–217. - PubMed
Publication types
MeSH terms
Substances
Associated data
- Actions
Grants and funding
LinkOut - more resources
Full Text Sources
Other Literature Sources
Molecular Biology Databases
Miscellaneous