Chaperone-mediated coupling of endoplasmic reticulum and mitochondrial Ca2+ channels
- PMID: 17178908
- PMCID: PMC2064700
- DOI: 10.1083/jcb.200608073
Chaperone-mediated coupling of endoplasmic reticulum and mitochondrial Ca2+ channels
Abstract
The voltage-dependent anion channel (VDAC) of the outer mitochondrial membrane mediates metabolic flow, Ca(2+), and cell death signaling between the endoplasmic reticulum (ER) and mitochondrial networks. We demonstrate that VDAC1 is physically linked to the endoplasmic reticulum Ca(2+)-release channel inositol 1,4,5-trisphosphate receptor (IP(3)R) through the molecular chaperone glucose-regulated protein 75 (grp75). Functional interaction between the channels was shown by the recombinant expression of the ligand-binding domain of the IP(3)R on the ER or mitochondrial surface, which directly enhanced Ca(2+) accumulation in mitochondria. Knockdown of grp75 abolished the stimulatory effect, highlighting chaperone-mediated conformational coupling between the IP(3)R and the mitochondrial Ca(2+) uptake machinery. Because organelle Ca(2+) homeostasis influences fundamentally cellular functions and death signaling, the central location of grp75 may represent an important control point of cell fate and pathogenesis.
Figures
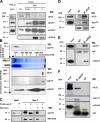
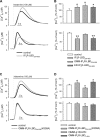
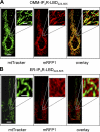
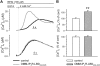
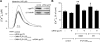
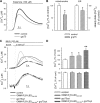
Similar articles
-
Reduction of Mitochondria-Endoplasmic Reticulum Interactions by Acetylcholine Protects Human Umbilical Vein Endothelial Cells From Hypoxia/Reoxygenation Injury.Arterioscler Thromb Vasc Biol. 2015 Jul;35(7):1623-34. doi: 10.1161/ATVBAHA.115.305469. Epub 2015 May 14. Arterioscler Thromb Vasc Biol. 2015. PMID: 25977565
-
A trio has turned into a quartet: DJ-1 interacts with the IP3R-Grp75-VDAC complex to control ER-mitochondria interaction.Cell Calcium. 2020 May;87:102186. doi: 10.1016/j.ceca.2020.102186. Epub 2020 Feb 24. Cell Calcium. 2020. PMID: 32120195
-
Tespa1 is a novel component of mitochondria-associated endoplasmic reticulum membranes and affects mitochondrial calcium flux.Biochem Biophys Res Commun. 2013 Apr 12;433(3):322-6. doi: 10.1016/j.bbrc.2013.02.099. Epub 2013 Mar 15. Biochem Biophys Res Commun. 2013. PMID: 23501103
-
The ER-mitochondria interface, where Ca2+ and cell death meet.Cell Calcium. 2023 Jun;112:102743. doi: 10.1016/j.ceca.2023.102743. Epub 2023 Apr 25. Cell Calcium. 2023. PMID: 37126911 Review.
-
Cypermethrin induces Sertoli cell apoptosis through endoplasmic reticulum-mitochondrial coupling involving IP3R1-GRP75-VDAC1.Reprod Toxicol. 2024 Mar;124:108552. doi: 10.1016/j.reprotox.2024.108552. Epub 2024 Jan 29. Reprod Toxicol. 2024. PMID: 38296003 Review.
Cited by
-
An interaction between Bcl-xL and the voltage-dependent anion channel (VDAC) promotes mitochondrial Ca2+ uptake.J Biol Chem. 2013 Jul 5;288(27):19870-81. doi: 10.1074/jbc.M112.448290. Epub 2013 May 17. J Biol Chem. 2013. PMID: 23720737 Free PMC article.
-
Endoplasmic Reticulum-Mitochondria Contact Sites-Emerging Intracellular Signaling Hubs.Front Cell Dev Biol. 2021 May 20;9:653828. doi: 10.3389/fcell.2021.653828. eCollection 2021. Front Cell Dev Biol. 2021. PMID: 34095118 Free PMC article. Review.
-
Morphological control of mitochondrial bioenergetics.Front Biosci (Landmark Ed). 2015 Jan 1;20(2):229-46. doi: 10.2741/4306. Front Biosci (Landmark Ed). 2015. PMID: 25553448 Free PMC article. Review.
-
Stress responses from the endoplasmic reticulum in cancer.Front Oncol. 2015 Apr 20;5:93. doi: 10.3389/fonc.2015.00093. eCollection 2015. Front Oncol. 2015. PMID: 25941664 Free PMC article. Review.
-
miR-29a differentially regulates cell survival in astrocytes from cornu ammonis 1 and dentate gyrus by targeting VDAC1.Mitochondrion. 2016 Sep;30:248-54. doi: 10.1016/j.mito.2016.08.013. Epub 2016 Aug 21. Mitochondrion. 2016. PMID: 27553862 Free PMC article.
References
-
- Berridge, M.J., M.D. Bootman, and H.L. Roderick. 2003. Calcium signalling: dynamics, homeostasis and remodelling. Nat. Rev. Mol. Cell Biol. 4:517–529. - PubMed
-
- Bosanac, I., T. Michikawa, and K. Mikoshiba. 2004. Structural insights into the regulatory mechanism of IP3 receptor. Biochim. Biophys. Acta. 1742:89–102. - PubMed
Publication types
MeSH terms
Substances
Grants and funding
LinkOut - more resources
Full Text Sources
Other Literature Sources
Miscellaneous