Txk, a member of the non-receptor tyrosine kinase of the Tec family, forms a complex with poly(ADP-ribose) polymerase 1 and elongation factor 1alpha and regulates interferon-gamma gene transcription in Th1 cells
- PMID: 17177976
- PMCID: PMC1810450
- DOI: 10.1111/j.1365-2249.2006.03249.x
Txk, a member of the non-receptor tyrosine kinase of the Tec family, forms a complex with poly(ADP-ribose) polymerase 1 and elongation factor 1alpha and regulates interferon-gamma gene transcription in Th1 cells
Abstract
We have found previously that Txk, a member of the Tec family tyrosine kinases, is involved importantly in T helper 1 (Th1) cytokine production. However, how Txk regulates interferon (IFN)-gamma gene transcription in human T lymphocytes was not fully elucidated. In this study, we identified poly(ADP-ribose) polymerase 1 (PARP1) and elongation factor 1alpha (EF-1alpha) as Txk-associated molecules that bound to the Txk responsive element of the IFN-gamma gene promoter. Txk phosphorylated EF-1alpha and PARP1 formed a complex with them, and bound to the IFN-gamma gene promoter in vitro. In particular, the N terminal region containing the DNA binding domain of PARP1 was important for the trimolecular complex formation involving Txk, EF-1alpha and PARP1. Several mutant Txk which lacked kinase activity were unable to form the trimolecular complex. A PARP1 inhibitor, PJ34, suppressed IFN-gamma but not interleukin (IL)-4 production by normal peripheral blood lymphocytes (PBL). Multi-colour confocal analysis revealed that Txk and EF-1alpha located in the cytoplasm in the resting condition. Upon activation, a complex involving Txk, EF-1alpha and PARP1 was formed and was located in the nucleus. Collectively, Txk in combination with EF-1alpha and PARP1 bound to the IFN-gamma gene promoter, and exerted transcriptional activity on the IFN-gamma gene.
Figures
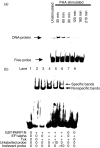
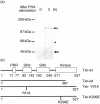
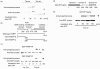
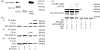
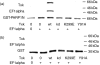
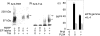
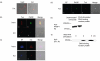
Similar articles
-
Txk, a member of nonreceptor tyrosine kinase of Tec family, acts as a Th1 cell-specific transcription factor and regulates IFN-gamma gene transcription.J Immunol. 2002 Mar 1;168(5):2365-70. doi: 10.4049/jimmunol.168.5.2365. J Immunol. 2002. PMID: 11859127
-
Txk, a nonreceptor tyrosine kinase of the Tec family, is expressed in T helper type 1 cells and regulates interferon gamma production in human T lymphocytes.J Exp Med. 1999 Oct 18;190(8):1147-54. doi: 10.1084/jem.190.8.1147. J Exp Med. 1999. PMID: 10523612 Free PMC article.
-
Role of Txk, a member of the Tec family of tyrosine kinases, in immune-inflammatory diseases.Int Rev Immunol. 2007 Sep-Dec;26(5-6):333-48. doi: 10.1080/08830180701690835. Int Rev Immunol. 2007. PMID: 18027204 Review.
-
Evidence of autophosphorylation in Txk: Y91 is an autophosphorylation site.Biol Pharm Bull. 2002 Jun;25(6):718-21. doi: 10.1248/bpb.25.718. Biol Pharm Bull. 2002. PMID: 12081135
-
Skewed Th1 responses caused by excessive expression of Txk, a member of the Tec family of tyrosine kinases, in patients with Behcet's disease.Clin Med Res. 2006 Jun;4(2):147-51. doi: 10.3121/cmr.4.2.147. Clin Med Res. 2006. PMID: 16809408 Free PMC article. Review.
Cited by
-
Co-expression of RelA/p65 and ACTN4 induces apoptosis in non-small lung carcinoma cells.Cell Cycle. 2018;17(5):616-626. doi: 10.1080/15384101.2017.1417709. Epub 2018 Jan 22. Cell Cycle. 2018. PMID: 29251177 Free PMC article.
-
Transcriptome Analysis of the Cerebellum of Mice Fed a Manganese-Deficient Diet.Front Genet. 2020 Dec 3;11:558725. doi: 10.3389/fgene.2020.558725. eCollection 2020. Front Genet. 2020. PMID: 33408735 Free PMC article.
-
c-Abl-Mediated Tyrosine Phosphorylation of PARP1 Is Crucial for Expression of Proinflammatory Genes.J Immunol. 2019 Sep 15;203(6):1521-1531. doi: 10.4049/jimmunol.1801616. Epub 2019 Aug 9. J Immunol. 2019. PMID: 31399520 Free PMC article.
-
Signaling mechanism of poly(ADP-ribose) polymerase-1 (PARP-1) in inflammatory diseases.Am J Pathol. 2011 Mar;178(3):946-55. doi: 10.1016/j.ajpath.2010.12.004. Am J Pathol. 2011. PMID: 21356345 Free PMC article. Review.
-
Expression and Clinical Value of Eukaryotic Translation Elongation Factor 1A1 (EEF1A1) in Diffuse Large B Cell Lymphoma.Int J Gen Med. 2021 Oct 27;14:7247-7258. doi: 10.2147/IJGM.S324645. eCollection 2021. Int J Gen Med. 2021. PMID: 34737619 Free PMC article.
References
-
- Szabo SJ, Kim ST, Costa GL, Zhang X, Fathman CG, Glimcher LH. A novel transcription factor, T-bet, directs Th1 lineage commitment. Cell. 2000;100:655–69. - PubMed
-
- Mullen AC, Hutchins AS, High FA, et al. Hlx is induced by and genetically interacts with T-bet to promote heritable T(H)1 gene induction. Nat Immunol. 2002;3:652–8. - PubMed
-
- Zheng W, Flavell RA. The transcription factor GATA-3 is necessary and sufficient for Th2 cytokine gene expression in CD4 T cells. Cell. 1997;89:587–96. - PubMed
-
- Messi M, Giacchetto I, Nagata K, Lanzavecchia A, Natoli G, Sallusto F. Memory and flexibility of cytokine gene expression as separable properties of human T(H)1 and T(H)2 lymphocytes. Nat Immunol. 2003;4:78–86. - PubMed
MeSH terms
Substances
LinkOut - more resources
Full Text Sources
Molecular Biology Databases
Miscellaneous