Phosphorylation and activity of the tumor suppressor Merlin and the ERM protein Moesin are coordinately regulated by the Slik kinase
- PMID: 17060498
- PMCID: PMC2064571
- DOI: 10.1083/jcb.200608009
Phosphorylation and activity of the tumor suppressor Merlin and the ERM protein Moesin are coordinately regulated by the Slik kinase
Abstract
Merlin and Moesin are closely related members of the 4.1 Ezrin/Radixin/Moesin domain superfamily implicated in regulating proliferation and epithelial integrity, respectively. The activity of both proteins is regulated by head to tail folding that is controlled, in part, by phosphorylation. Few upstream regulators of these phosphorylation events are known. In this study, we demonstrate that in Drosophila melanogaster, Slik, a Ste20 kinase, controls subcellular localization and phosphorylation of Merlin, resulting in the coordinate but opposite regulation of Merlin and Moesin. These results suggest the existence of a novel mechanism for coordinate regulation of cell proliferation and epithelial integrity in developing tissues.
Figures
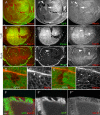
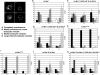
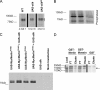
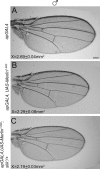
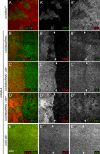
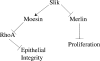
Similar articles
-
The PP1 phosphatase flapwing regulates the activity of Merlin and Moesin in Drosophila.Dev Biol. 2012 Jan 15;361(2):412-26. doi: 10.1016/j.ydbio.2011.11.007. Epub 2011 Nov 19. Dev Biol. 2012. PMID: 22133918
-
Regulation of Catalytic and Non-catalytic Functions of the Drosophila Ste20 Kinase Slik by Activation Segment Phosphorylation.J Biol Chem. 2015 Aug 21;290(34):20960-20971. doi: 10.1074/jbc.M115.645952. Epub 2015 Jul 13. J Biol Chem. 2015. PMID: 26170449 Free PMC article.
-
STRIPAK regulates Slik localization to control mitotic morphogenesis and epithelial integrity.J Cell Biol. 2020 Nov 2;219(11):e201911035. doi: 10.1083/jcb.201911035. J Cell Biol. 2020. PMID: 32960945 Free PMC article.
-
Inactivation of Merlin in malignant mesothelioma cells and the Hippo signaling cascade dysregulation.Pathol Int. 2011 Jun;61(6):331-44. doi: 10.1111/j.1440-1827.2011.02666.x. Epub 2011 May 2. Pathol Int. 2011. PMID: 21615608 Review.
-
Two Sides of the Coin: Ezrin/Radixin/Moesin and Merlin Control Membrane Structure and Contact Inhibition.Int J Mol Sci. 2019 Apr 23;20(8):1996. doi: 10.3390/ijms20081996. Int J Mol Sci. 2019. PMID: 31018575 Free PMC article. Review.
Cited by
-
CCM3 signaling through sterile 20-like kinases plays an essential role during zebrafish cardiovascular development and cerebral cavernous malformations.J Clin Invest. 2010 Aug;120(8):2795-804. doi: 10.1172/JCI39679. Epub 2010 Jul 1. J Clin Invest. 2010. PMID: 20592472 Free PMC article.
-
Conundrum, an ARHGAP18 orthologue, regulates RhoA and proliferation through interactions with Moesin.Mol Biol Cell. 2013 May;24(9):1420-33. doi: 10.1091/mbc.E12-11-0800. Epub 2013 Mar 6. Mol Biol Cell. 2013. PMID: 23468526 Free PMC article.
-
In vivo functional analysis of the human NF2 tumor suppressor gene in Drosophila.PLoS One. 2014 Mar 4;9(3):e90853. doi: 10.1371/journal.pone.0090853. eCollection 2014. PLoS One. 2014. PMID: 24595234 Free PMC article.
-
Microtubule-mediated transport of the tumor-suppressor protein Merlin and its mutants.Proc Natl Acad Sci U S A. 2010 Apr 20;107(16):7311-6. doi: 10.1073/pnas.0907389107. Epub 2010 Apr 5. Proc Natl Acad Sci U S A. 2010. PMID: 20368450 Free PMC article.
-
Moesin and its activating kinase Slik are required for cortical stability and microtubule organization in mitotic cells.J Cell Biol. 2008 Feb 25;180(4):739-46. doi: 10.1083/jcb.200709161. Epub 2008 Feb 18. J Cell Biol. 2008. PMID: 18283112 Free PMC article.
References
-
- Bilder, D. 2004. Epithelial polarity and proliferation control: links from the Drosophila neoplastic tumor suppressors. Genes Dev. 18:1909–1925. - PubMed
-
- Bilder, D., M. Li, and N. Perrimon. 2000. Cooperative regulation of cell polarity and growth by Drosophila tumor suppressors. Science. 289:113–116. - PubMed
-
- Brand, A.H., and N. Perrimon. 1993. Targeted gene expression as a means of altering cell fates and generating dominant phenotypes. Development. 118:401–415. - PubMed
-
- Bretscher, A., K. Edwards, and R.G. Fehon. 2002. ERM proteins and Merlin: integrators at the cell cortex. Nat. Rev. Mol. Cell Biol. 3:586–599. - PubMed
-
- Bryant, P.J., and P. Simpson. 1984. Intrinsic and extrinsic control of growth in developing organs. Q. Rev. Biol. 59:387–415. - PubMed
Publication types
MeSH terms
Substances
Grants and funding
LinkOut - more resources
Full Text Sources
Molecular Biology Databases
Research Materials