Manipulating Kv4.2 identifies a specific component of hippocampal pyramidal neuron A-current that depends upon Kv4.2 expression
- PMID: 17026528
- PMCID: PMC3583589
- DOI: 10.1111/j.1471-4159.2006.04185.x
Manipulating Kv4.2 identifies a specific component of hippocampal pyramidal neuron A-current that depends upon Kv4.2 expression
Abstract
The somatodendritic A-current, I(SA), in hippocampal CA1 pyramidal neurons regulates the processing of synaptic inputs and the amplitude of back propagating action potentials into the dendritic tree, as well as the action potential firing properties at the soma. In this study, we have used RNA interference and over-expression to show that expression of the Kv4.2 gene specifically regulates the I(SA) component of A-current in these neurons. In dissociated hippocampal pyramidal neuron cultures, or organotypic cultured CA1 pyramidal neurons, the expression level of Kv4.2 is such that the I(SA) channels are maintained in the population at a peak conductance of approximately 950 pS/pF. Suppression of Kv4.2 transcripts in hippocampal pyramidal neurons using an RNA interference vector suppresses I(SA) current by 60% in 2 days, similar to the effect of expressing dominant-negative Kv4 channel constructs. Increasing the expression of Kv4.2 in these neurons increases the level of I(SA) to 170% of the normal set point without altering the biophysical properties. Our results establish a specific role for native Kv4.2 transcripts in forming and maintaining I(SA) current at characteristic levels in hippocampal pyramidal neurons.
Figures
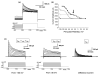
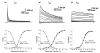
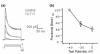
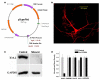
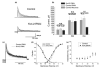
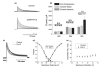
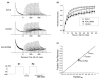
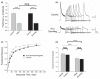
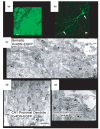
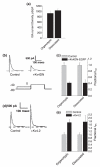
Similar articles
-
Rapid, bidirectional remodeling of synaptic NMDA receptor subunit composition by A-type K+ channel activity in hippocampal CA1 pyramidal neurons.Neuron. 2008 Nov 26;60(4):657-71. doi: 10.1016/j.neuron.2008.08.029. Neuron. 2008. PMID: 19038222 Free PMC article.
-
Differential cycling rates of Kv4.2 channels in proximal and distal dendrites of hippocampal CA1 pyramidal neurons.Hippocampus. 2012 May;22(5):969-80. doi: 10.1002/hipo.20899. Epub 2011 Apr 6. Hippocampus. 2012. PMID: 21472817 Free PMC article.
-
Dendritic localization and a cis-acting dendritic targeting element of Kv4.2 mRNA.BMB Rep. 2010 Oct;43(10):677-82. doi: 10.5483/BMBRep.2010.43.10.677. BMB Rep. 2010. PMID: 21034530
-
Altered synaptic and non-synaptic properties of CA1 pyramidal neurons in Kv4.2 knockout mice.J Physiol. 2008 Aug 15;586(16):3881-92. doi: 10.1113/jphysiol.2008.154336. Epub 2008 Jun 19. J Physiol. 2008. PMID: 18566000 Free PMC article.
-
Dendritic potassium channels in hippocampal pyramidal neurons.J Physiol. 2000 May 15;525 Pt 1(Pt 1):75-81. doi: 10.1111/j.1469-7793.2000.00075.x. J Physiol. 2000. PMID: 10811726 Free PMC article. Review.
Cited by
-
Localization and targeting of voltage-dependent ion channels in mammalian central neurons.Physiol Rev. 2008 Oct;88(4):1407-47. doi: 10.1152/physrev.00002.2008. Physiol Rev. 2008. PMID: 18923186 Free PMC article. Review.
-
S3b amino acid substitutions and ancillary subunits alter the affinity of Heteropoda venatoria toxin 2 for Kv4.3.Mol Pharmacol. 2009 Jul;76(1):125-33. doi: 10.1124/mol.109.055657. Epub 2009 Apr 8. Mol Pharmacol. 2009. PMID: 19357248 Free PMC article.
-
Potassium channels: newly found players in synaptic plasticity.Neuroscientist. 2008 Jun;14(3):276-86. doi: 10.1177/1073858408315041. Epub 2008 Apr 15. Neuroscientist. 2008. PMID: 18413784 Free PMC article. Review.
-
Prominent hippocampal CA3 gene expression profile in neurocognitive aging.Neurobiol Aging. 2011 Sep;32(9):1678-92. doi: 10.1016/j.neurobiolaging.2009.10.005. Epub 2009 Nov 13. Neurobiol Aging. 2011. PMID: 19913943 Free PMC article.
-
Dipeptidyl peptidase-like protein 6 is required for normal electrophysiological properties of cerebellar granule cells.J Neurosci. 2010 Jun 23;30(25):8551-65. doi: 10.1523/JNEUROSCI.5489-09.2010. J Neurosci. 2010. PMID: 20573902 Free PMC article.
References
-
- An WF, Bowlby MR, Betty M, et al. Modulation of A-type potassium channels by a family of calcium sensors. Nature. 2000;403:553–556. - PubMed
-
- Bahring R, Dannenberg J, Peters HC, Leicher T, Pongs O, Isbrandt D. Conserved Kv4 N-terminal domain critical for effects of Kv channel-interacting protein 2.2 on channel expression and gating. J. Biol. Chem. 2001;276:23 888–23 894. - PubMed
-
- Baldwin TJ, Tsaur ML, Lopez GA, Jan YN, Jan LY. Characterization of a mammalian cDNA for an inactivating voltage-sensitive K+ channel. Neuron. 1991;7:471–483. - PubMed
-
- Barry DM, Xu H, Schuessler RB, Nerbonne JM. Functional knockout of the transient outward current, long-QT syndrome, and cardiac remodeling in mice expressing a dominant-negative Kv4α subunit. Circ. Res. 1998;83:560–567. - PubMed
Publication types
MeSH terms
Substances
Grants and funding
LinkOut - more resources
Full Text Sources
Molecular Biology Databases
Miscellaneous