Thyrotropin-releasing hormone increases GABA release in rat hippocampus
- PMID: 16990402
- PMCID: PMC1890442
- DOI: 10.1113/jphysiol.2006.118141
Thyrotropin-releasing hormone increases GABA release in rat hippocampus
Abstract
Thyrotropin-releasing hormone (TRH) is a tripeptide that is widely distributed in the brain including the hippocampus where TRH receptors are also expressed. TRH has anti-epileptic effects and regulates arousal, sleep, cognition, locomotion and mood. However, the cellular mechanisms underlying such effects remain to be determined. We examined the effects of TRH on GABAergic transmission in the hippocampus and found that TRH increased the frequency of GABAA receptor-mediated spontaneous IPSCs in each region of the hippocampus but had no effects on miniature IPSCs or evoked IPSCs. TRH increased the action potential firing frequency recorded from GABAergic interneurons in CA1 stratum radiatum and induced membrane depolarization suggesting that TRH increases the excitability of interneurons to facilitate GABA release. TRH-induced inward current had a reversal potential close to the K+ reversal potential suggesting that TRH inhibits resting K+ channels. The involved K+ channels were sensitive to Ba2+ but resistant to other classical K+ channel blockers, suggesting that TRH inhibits the two-pore domain K+ channels. Because the effects of TRH were mediated via Galphaq/11, but were independent of its known downstream effectors, a direct coupling may exist between Galphaq/11 and K+ channels. Inhibition of the function of dynamin slowed the desensitization of TRH responses. TRH inhibited seizure activity induced by Mg2+ deprivation, but not that generated by picrotoxin, suggesting that TRH-mediated increase in GABA release contributes to its anti-epileptic effects. Our results demonstrate a novel mechanism to explain some of the hippocampal actions of TRH.
Figures
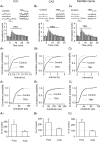
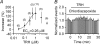
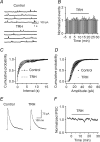
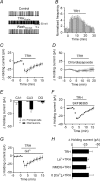
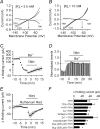
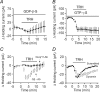
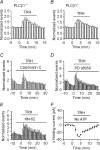
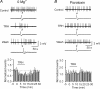
Similar articles
-
Vasopressin facilitates GABAergic transmission in rat hippocampus via activation of V(1A) receptors.Neuropharmacology. 2012 Dec;63(7):1218-26. doi: 10.1016/j.neuropharm.2012.07.043. Epub 2012 Aug 3. Neuropharmacology. 2012. PMID: 22884625 Free PMC article.
-
Bidirectional modulation of GABAergic transmission by cholecystokinin in hippocampal dentate gyrus granule cells of juvenile rats.J Physiol. 2006 Apr 15;572(Pt 2):425-42. doi: 10.1113/jphysiol.2005.104463. Epub 2006 Feb 2. J Physiol. 2006. PMID: 16455686 Free PMC article.
-
In the developing rat hippocampus a tonic GABAA-mediated conductance selectively enhances the glutamatergic drive of principal cells.J Physiol. 2007 Jun 1;581(Pt 2):515-28. doi: 10.1113/jphysiol.2006.125609. Epub 2007 Feb 22. J Physiol. 2007. PMID: 17317750 Free PMC article.
-
Cell type specificity of GABA(A) receptor mediated signaling in the hippocampus.Curr Drug Targets CNS Neurol Disord. 2003 Aug;2(4):240-7. doi: 10.2174/1568007033482832. Curr Drug Targets CNS Neurol Disord. 2003. PMID: 12871034 Review.
-
Hippocampal GABAergic Inhibitory Interneurons.Physiol Rev. 2017 Oct 1;97(4):1619-1747. doi: 10.1152/physrev.00007.2017. Physiol Rev. 2017. PMID: 28954853 Free PMC article. Review.
Cited by
-
Cholecystokinin facilitates glutamate release by increasing the number of readily releasable vesicles and releasing probability.J Neurosci. 2010 Apr 14;30(15):5136-48. doi: 10.1523/JNEUROSCI.5711-09.2010. J Neurosci. 2010. PMID: 20392936 Free PMC article.
-
Roles of K+ and cation channels in ORL-1 receptor-mediated depression of neuronal excitability and epileptic activities in the medial entorhinal cortex.Neuropharmacology. 2019 Jun;151:144-158. doi: 10.1016/j.neuropharm.2019.04.017. Epub 2019 Apr 15. Neuropharmacology. 2019. PMID: 30998945 Free PMC article.
-
Vasopressin facilitates GABAergic transmission in rat hippocampus via activation of V(1A) receptors.Neuropharmacology. 2012 Dec;63(7):1218-26. doi: 10.1016/j.neuropharm.2012.07.043. Epub 2012 Aug 3. Neuropharmacology. 2012. PMID: 22884625 Free PMC article.
-
Increased EID1 nuclear translocation impairs synaptic plasticity and memory function associated with pathogenesis of Alzheimer's disease.Neurobiol Dis. 2012 Mar;45(3):902-12. doi: 10.1016/j.nbd.2011.12.007. Epub 2011 Dec 11. Neurobiol Dis. 2012. PMID: 22186421 Free PMC article.
-
The role of Thyrotropin Releasing Hormone in aging and neurodegenerative diseases.Am J Alzheimers Dis (Columbia). 2013;1(1):10.7726/ajad.2013.1003. doi: 10.7726/ajad.2013.1003. Am J Alzheimers Dis (Columbia). 2013. PMID: 24199031 Free PMC article.
References
-
- Bannister RA, Melliti K, Adams BA. Differential modulation of CaV2.3 Ca2+ channels by Gαq/11-coupled muscarinic receptors. Mol Pharmacol. 2004;65:381–388. - PubMed
-
- Bayliss DA, Sirois JE, Talley EM. The TASK family: two-pore domain background K+ channels. Mol Interv. 2003;3:205–219. - PubMed
-
- Bayliss DA, Viana F, Berger AJ. Mechanisms underlying excitatory effects of thyrotropin-releasing hormone on rat hypoglossal motoneurons in vitro. J Neurophysiol. 1992;68:1733–1745. - PubMed
-
- Bayliss DA, Viana F, Berger AJ. Effects of thyrotropin-releasing hormone on rat motoneurons are mediated by G proteins. Brain Res. 1994;668:220–229. - PubMed
Publication types
MeSH terms
Substances
Grants and funding
LinkOut - more resources
Full Text Sources
Molecular Biology Databases
Miscellaneous