Different routes of bone morphogenic protein (BMP) receptor endocytosis influence BMP signaling
- PMID: 16923969
- PMCID: PMC1636853
- DOI: 10.1128/MCB.00022-06
Different routes of bone morphogenic protein (BMP) receptor endocytosis influence BMP signaling
Abstract
Endocytosis is important for a variety of functions in eukaryotic cells, including the regulation of signaling cascades via transmembrane receptors. The internalization of bone morphogenetic protein (BMP) receptor type I (BRI) and type II (BRII) and its relation to signaling were largely unexplored. Here, we demonstrate that both receptor types undergo constitutive endocytosis via clathrin-coated pits (CCPs) but that only BRII undergoes also caveola-like internalization. Using several complementary approaches, we could show that (i) BMP-2-mediated Smad1/5 phosphorylation occurs at the plasma membrane in nonraft regions, (ii) continuation of Smad signaling resulting in a transcriptional response requires endocytosis via the clathrin-mediated route, and (iii) BMP signaling leading to alkaline phosphatase induction initiates from receptors that fractionate into cholesterol-enriched, detergent-resistant membranes. Furthermore, we show that BRII interacts with Eps15R, a constitutive component of CCPs, and with caveolin-1, the marker protein of caveolae. Taken together, the localization of BMP receptors in distinct membrane domains is prerequisite to their taking different endocytosis routes with specific impacts on Smad-dependent and Smad-independent signaling cascades.
Figures
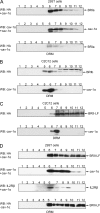
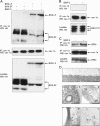
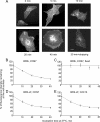
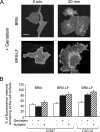
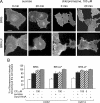
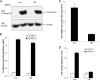
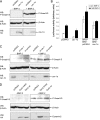
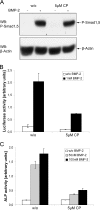
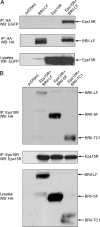
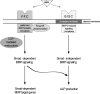
Similar articles
-
Dynamics and interaction of caveolin-1 isoforms with BMP-receptors.J Cell Sci. 2005 Feb 1;118(Pt 3):643-50. doi: 10.1242/jcs.01402. Epub 2005 Jan 18. J Cell Sci. 2005. PMID: 15657086
-
Mutations in bone morphogenetic protein type II receptor cause dysregulation of Id gene expression in pulmonary artery smooth muscle cells: implications for familial pulmonary arterial hypertension.Circ Res. 2008 May 23;102(10):1212-21. doi: 10.1161/CIRCRESAHA.108.173567. Epub 2008 Apr 24. Circ Res. 2008. PMID: 18436795
-
Endofin acts as a Smad anchor for receptor activation in BMP signaling.J Cell Sci. 2007 Apr 1;120(Pt 7):1216-24. doi: 10.1242/jcs.03400. Epub 2007 Mar 13. J Cell Sci. 2007. PMID: 17356069
-
Recent advances in BMP receptor signaling.Cytokine Growth Factor Rev. 2009 Oct-Dec;20(5-6):343-55. doi: 10.1016/j.cytogfr.2009.10.007. Epub 2009 Nov 7. Cytokine Growth Factor Rev. 2009. PMID: 19897402 Review.
-
Membrane microdomains, caveolae, and caveolar endocytosis of sphingolipids.Mol Membr Biol. 2006 Jan-Feb;23(1):101-10. doi: 10.1080/09687860500460041. Mol Membr Biol. 2006. PMID: 16611585 Review.
Cited by
-
Elafin Reverses Pulmonary Hypertension via Caveolin-1-Dependent Bone Morphogenetic Protein Signaling.Am J Respir Crit Care Med. 2015 Jun 1;191(11):1273-86. doi: 10.1164/rccm.201412-2291OC. Am J Respir Crit Care Med. 2015. PMID: 25853696 Free PMC article.
-
Atheroprone fluid shear stress-regulated ALK1-Endoglin-SMAD signaling originates from early endosomes.BMC Biol. 2022 Sep 28;20(1):210. doi: 10.1186/s12915-022-01396-y. BMC Biol. 2022. PMID: 36171573 Free PMC article.
-
The lysosomal inhibitor, chloroquine, increases cell surface BMPR-II levels and restores BMP9 signalling in endothelial cells harbouring BMPR-II mutations.Hum Mol Genet. 2013 Sep 15;22(18):3667-79. doi: 10.1093/hmg/ddt216. Epub 2013 May 12. Hum Mol Genet. 2013. PMID: 23669347 Free PMC article.
-
Visualization of BRI1 and BAK1(SERK3) membrane receptor heterooligomers during brassinosteroid signaling.Plant Physiol. 2013 Aug;162(4):1911-25. doi: 10.1104/pp.113.220152. Epub 2013 Jun 24. Plant Physiol. 2013. PMID: 23796795 Free PMC article.
-
Transferrin-directed internalization and cycling of transferrin receptor 2.Traffic. 2009 Oct;10(10):1488-501. doi: 10.1111/j.1600-0854.2009.00961.x. Epub 2009 Jul 6. Traffic. 2009. PMID: 19682329 Free PMC article.
References
-
- Anderson, R. G. 1998. The caveolae membrane system. Annu. Rev. Biochem. 67:199-225. - PubMed
-
- Anderson, R. G., and K. Jacobson. 2002. A role for lipid shells in targeting proteins to caveolae, rafts, and other lipid domains. Science 296:1821-1825. - PubMed
-
- Anderson, R. G., B. A. Kamen, K. G. Rothberg, and S. W. Lacey. 1992. Potocytosis: sequestration and transport of small molecules by caveolae. Science 255:410-411. - PubMed
-
- Aoki, T., R. Nomura, and T. Fujimoto. 1999. Tyrosine phosphorylation of caveolin-1 in the endothelium. Exp. Cell Res. 253:629-636. - PubMed
-
- Brown, D. A., and E. London. 2000. Structure and function of sphingolipid- and cholesterol-rich membrane rafts. J. Biol. Chem. 275:17221-17224. - PubMed
Publication types
MeSH terms
Substances
LinkOut - more resources
Full Text Sources
Other Literature Sources
Molecular Biology Databases