Vascular endothelial cadherin controls VEGFR-2 internalization and signaling from intracellular compartments
- PMID: 16893970
- PMCID: PMC2064264
- DOI: 10.1083/jcb.200602080
Vascular endothelial cadherin controls VEGFR-2 internalization and signaling from intracellular compartments
Abstract
Receptor endocytosis is a fundamental step in controlling the magnitude, duration, and nature of cell signaling events. Confluent endothelial cells are contact inhibited in their growth and respond poorly to the proliferative signals of vascular endothelial growth factor (VEGF). In a previous study, we found that the association of vascular endothelial cadherin (VEC) with VEGF receptor (VEGFR) type 2 contributes to density-dependent growth inhibition (Lampugnani, G.M., A. Zanetti, M. Corada, T. Takahashi, G. Balconi, F. Breviario, F. Orsenigo, A. Cattelino, R. Kemler, T.O. Daniel, and E. Dejana. 2003. J. Cell Biol. 161:793-804). In the present study, we describe the mechanism through which VEC reduces VEGFR-2 signaling. We found that VEGF induces the clathrin-dependent internalization of VEGFR-2. When VEC is absent or not engaged at junctions, VEGFR-2 is internalized more rapidly and remains in endosomal compartments for a longer time. Internalization does not terminate its signaling; instead, the internalized receptor is phosphorylated, codistributes with active phospholipase C-gamma, and activates p44/42 mitogen-activated protein kinase phosphorylation and cell proliferation. Inhibition of VEGFR-2 internalization reestablishes the contact inhibition of cell growth, whereas silencing the junction-associated density-enhanced phosphatase-1/CD148 phosphatase restores VEGFR-2 internalization and signaling. Thus, VEC limits cell proliferation by retaining VEGFR-2 at the membrane and preventing its internalization into signaling compartments.
Figures
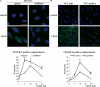
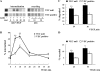
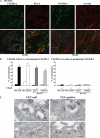
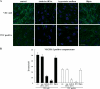
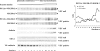
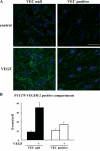
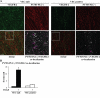
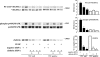
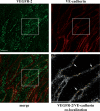
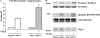
Similar articles
-
Contact inhibition of VEGF-induced proliferation requires vascular endothelial cadherin, beta-catenin, and the phosphatase DEP-1/CD148.J Cell Biol. 2003 May 26;161(4):793-804. doi: 10.1083/jcb.200209019. J Cell Biol. 2003. PMID: 12771128 Free PMC article.
-
Vascular endothelial growth factor-induced endothelial cell proliferation is regulated by interaction between VEGFR-2, SH-PTP1 and eNOS.Microvasc Res. 2006 Jan;71(1):20-31. doi: 10.1016/j.mvr.2005.10.004. Epub 2005 Dec 9. Microvasc Res. 2006. PMID: 16337972
-
Role of protein tyrosine phosphatase 1B in vascular endothelial growth factor signaling and cell-cell adhesions in endothelial cells.Circ Res. 2008 May 23;102(10):1182-91. doi: 10.1161/CIRCRESAHA.107.167080. Epub 2008 May 1. Circ Res. 2008. PMID: 18451337 Free PMC article.
-
Vascular endothelial growth factor receptors: molecular mechanisms of activation and therapeutic potentials.Exp Eye Res. 2006 Nov;83(5):1005-16. doi: 10.1016/j.exer.2006.03.019. Epub 2006 May 19. Exp Eye Res. 2006. PMID: 16713597 Free PMC article. Review.
-
Vesicular trafficking of tyrosine kinase receptors and associated proteins in the regulation of signaling and vascular function.Circ Res. 2006 Mar 31;98(6):743-56. doi: 10.1161/01.RES.0000214545.99387.e3. Circ Res. 2006. PMID: 16574915 Review.
Cited by
-
Oxidative Stress Induces a VEGF Autocrine Loop in the Retina: Relevance for Diabetic Retinopathy.Cells. 2020 Jun 11;9(6):1452. doi: 10.3390/cells9061452. Cells. 2020. PMID: 32545222 Free PMC article.
-
VEGF-A isoforms program differential VEGFR2 signal transduction, trafficking and proteolysis.Biol Open. 2016 May 15;5(5):571-83. doi: 10.1242/bio.017434. Biol Open. 2016. PMID: 27044325 Free PMC article.
-
Mechanisms and regulation of endothelial VEGF receptor signalling.Nat Rev Mol Cell Biol. 2016 Oct;17(10):611-25. doi: 10.1038/nrm.2016.87. Epub 2016 Jul 27. Nat Rev Mol Cell Biol. 2016. PMID: 27461391 Review.
-
An inside view: VEGF receptor trafficking and signaling.Physiology (Bethesda). 2012 Aug;27(4):213-22. doi: 10.1152/physiol.00016.2012. Physiology (Bethesda). 2012. PMID: 22875452 Free PMC article. Review.
-
Nitric Oxide Synthase-2-Derived Nitric Oxide Drives Multiple Pathways of Breast Cancer Progression.Antioxid Redox Signal. 2017 Jun 20;26(18):1044-1058. doi: 10.1089/ars.2016.6813. Epub 2016 Sep 7. Antioxid Redox Signal. 2017. PMID: 27464521 Free PMC article. Review.
References
-
- Alitalo, K., T. Tammela, and T.V. Petrova. 2005. Lymphangiogenesis in development and human disease. Nature. 438:946–953. - PubMed
-
- Andriopoulou, P., P. Navarro, A. Zanetti, M.G. Lampugnani, and E. Dejana. 1999. Histamine induces tyrosine phosphorylation of endothelial cell-to-cell adherens junctions. Arterioscler. Thromb. Vasc. Biol. 19:2286– 2297. - PubMed
Publication types
MeSH terms
Substances
Grants and funding
LinkOut - more resources
Full Text Sources
Other Literature Sources
Molecular Biology Databases
Miscellaneous