Mannose receptor regulates myoblast motility and muscle growth
- PMID: 16864654
- PMCID: PMC2064236
- DOI: 10.1083/jcb.200601102
Mannose receptor regulates myoblast motility and muscle growth
Abstract
Myoblast fusion is critical for the formation, growth, and maintenance of skeletal muscle. The initial formation of nascent myotubes requires myoblast-myoblast fusion, but further growth involves myoblast-myotube fusion. We demonstrate that the mannose receptor (MR), a type I transmembrane protein, is required for myoblast-myotube fusion. Mannose receptor (MR)-null myotubes were small in size and contained a decreased myonuclear number both in vitro and in vivo. We hypothesized that this defect may arise from a possible role of MR in cell migration. Time-lapse microscopy revealed that MR-null myoblasts migrated with decreased velocity during myotube growth and were unable to migrate in a directed manner up a chemoattractant gradient. Furthermore, collagen uptake was impaired in MR-null myoblasts, suggesting a role in extracellular matrix remodeling during cell motility. These data identify a novel function for MR during skeletal muscle growth and suggest that myoblast motility may be a key aspect of regulating myotube growth.
Figures
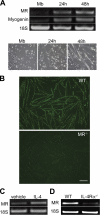
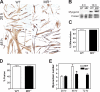
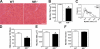
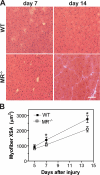
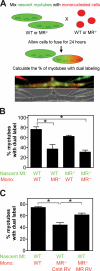
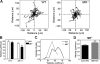
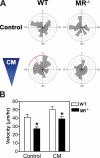
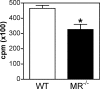
Similar articles
-
A novel in vitro model for the assessment of postnatal myonuclear accretion.Skelet Muscle. 2018 Feb 14;8(1):4. doi: 10.1186/s13395-018-0151-4. Skelet Muscle. 2018. PMID: 29444710 Free PMC article.
-
Phosphatidylserine receptor BAI1 and apoptotic cells as new promoters of myoblast fusion.Nature. 2013 May 9;497(7448):263-7. doi: 10.1038/nature12135. Epub 2013 Apr 24. Nature. 2013. PMID: 23615608 Free PMC article.
-
CD44 regulates myoblast migration and differentiation.J Cell Physiol. 2006 Nov;209(2):314-21. doi: 10.1002/jcp.20724. J Cell Physiol. 2006. PMID: 16906571
-
Mechanisms regulating myoblast fusion: A multilevel interplay.Semin Cell Dev Biol. 2020 Aug;104:81-92. doi: 10.1016/j.semcdb.2020.02.004. Epub 2020 Feb 13. Semin Cell Dev Biol. 2020. PMID: 32063453 Review.
-
The regulation of myoblast plasticity and its mechanism.Zhongguo Ying Yong Sheng Li Xue Za Zhi. 2012 Nov;28(6):524-31. Zhongguo Ying Yong Sheng Li Xue Za Zhi. 2012. PMID: 23581181 Review.
Cited by
-
Irradiation dependent inflammatory response may enhance satellite cell engraftment.Sci Rep. 2020 Jul 6;10(1):11119. doi: 10.1038/s41598-020-68098-9. Sci Rep. 2020. PMID: 32632224 Free PMC article.
-
Distinct actions of Akt1 and Akt2 in skeletal muscle differentiation.J Cell Physiol. 2009 May;219(2):503-11. doi: 10.1002/jcp.21692. J Cell Physiol. 2009. PMID: 19142853 Free PMC article.
-
The satellite cell in male and female, developing and adult mouse muscle: distinct stem cells for growth and regeneration.PLoS One. 2012;7(5):e37950. doi: 10.1371/journal.pone.0037950. Epub 2012 May 25. PLoS One. 2012. PMID: 22662253 Free PMC article.
-
In vitro myoblast motility models: investigating migration dynamics for the study of skeletal muscle repair.J Muscle Res Cell Motil. 2013 Dec;34(5-6):333-47. doi: 10.1007/s10974-013-9364-7. Epub 2013 Oct 23. J Muscle Res Cell Motil. 2013. PMID: 24150600 Review.
-
Myomaker, Regulated by MYOD, MYOG and miR-140-3p, Promotes Chicken Myoblast Fusion.Int J Mol Sci. 2015 Nov 2;16(11):26186-201. doi: 10.3390/ijms161125946. Int J Mol Sci. 2015. PMID: 26540045 Free PMC article.
References
-
- Allen, D.L., R.R. Roy, and V.R. Edgerton. 1999. Myonuclear domains in muscle adaptation and disease. Muscle Nerve. 22:1350–1360. - PubMed
-
- Birchmeier, C., and H. Brohmann. 2000. Genes that control the development of migrating muscle precursor cells. Curr. Opin. Cell Biol. 12:725–730. - PubMed
-
- Bischoff, R. 1997. Chemotaxis of skeletal muscle satellite cells. Dev. Dyn. 208:505–515. - PubMed
-
- Bondesen, B.A., S.T. Mills, K.M. Kegley, and G.K. Pavlath. 2004. The COX-2 pathway is essential during early stages of skeletal muscle regeneration. Am. J. Physiol. Cell Physiol. 287:C475–C483. - PubMed
Publication types
MeSH terms
Substances
Grants and funding
LinkOut - more resources
Full Text Sources
Other Literature Sources
Molecular Biology Databases