Synaptic plasticity deficits and mild memory impairments in mouse models of chronic granulomatous disease
- PMID: 16847341
- PMCID: PMC1592752
- DOI: 10.1128/MCB.00269-06
Synaptic plasticity deficits and mild memory impairments in mouse models of chronic granulomatous disease
Abstract
Reactive oxygen species (ROS) are required in a number of critical cellular signaling events, including those underlying hippocampal synaptic plasticity and hippocampus-dependent memory; however, the source of ROS is unknown. We previously have shown that NADPH oxidase is required for N-methyl-D-aspartate (NMDA) receptor-dependent signal transduction in the hippocampus, suggesting that NADPH oxidase may be required for NMDA receptor-dependent long-term potentiation (LTP) and hippocampus-dependent memory. Herein we present the first evidence that NADPH oxidase is involved in hippocampal synaptic plasticity and memory. We have found that pharmacological inhibitors of NADPH oxidase block LTP. Moreover, mice that lack the NADPH oxidase proteins gp91(phox) and p47(phox), both of which are mouse models of human chronic granulomatous disease (CGD), also lack LTP. We also found that the gp91(phox) and p47(phox) mutant mice have mild impairments in hippocampus-dependent memory. The gp91(phox) mutant mice exhibited a spatial memory deficit in the Morris water maze, and the p47(phox) mutant mice exhibited impaired context-dependent fear memory. Taken together, our results are consistent with NADPH oxidase being required for hippocampal synaptic plasticity and memory and are consistent with reports of cognitive dysfunction in patients with CGD.
Figures
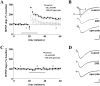
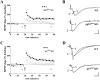
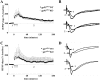
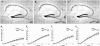
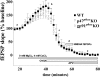
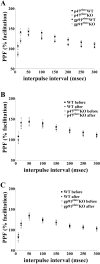
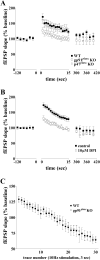
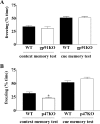

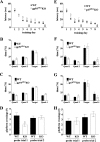

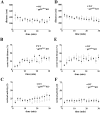
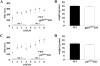
Similar articles
-
Transgenic mice expressing a truncated form of CREB-binding protein (CBP) exhibit deficits in hippocampal synaptic plasticity and memory storage.Learn Mem. 2005 Mar-Apr;12(2):111-9. doi: 10.1101/lm.86605. Learn Mem. 2005. PMID: 15805310 Free PMC article.
-
Hippocampal synaptic modulation by the phosphotyrosine adapter protein ShcC/N-Shc via interaction with the NMDA receptor.J Neurosci. 2005 Feb 16;25(7):1826-35. doi: 10.1523/JNEUROSCI.3030-04.2005. J Neurosci. 2005. PMID: 15716419 Free PMC article.
-
Glial protein S100B modulates long-term neuronal synaptic plasticity.Proc Natl Acad Sci U S A. 2002 Mar 19;99(6):4037-42. doi: 10.1073/pnas.052020999. Epub 2002 Mar 12. Proc Natl Acad Sci U S A. 2002. PMID: 11891290 Free PMC article.
-
Plasticity, hippocampal place cells, and cognitive maps.Arch Neurol. 2001 Jun;58(6):874-81. doi: 10.1001/archneur.58.6.874. Arch Neurol. 2001. PMID: 11405801 Review.
-
Hippocampal synaptic plasticity: role in spatial learning or the automatic recording of attended experience?Philos Trans R Soc Lond B Biol Sci. 1997 Oct 29;352(1360):1489-503. doi: 10.1098/rstb.1997.0136. Philos Trans R Soc Lond B Biol Sci. 1997. PMID: 9368938 Free PMC article. Review.
Cited by
-
NADPH oxidases: novel therapeutic targets for neurodegenerative diseases.Trends Pharmacol Sci. 2012 Jun;33(6):295-303. doi: 10.1016/j.tips.2012.03.008. Epub 2012 Apr 11. Trends Pharmacol Sci. 2012. PMID: 22503440 Free PMC article. Review.
-
Behavioral and Synaptic Phenotypes of Female Prdx6-/- Mice.Antioxidants (Basel). 2022 Jun 19;11(6):1201. doi: 10.3390/antiox11061201. Antioxidants (Basel). 2022. PMID: 35740098 Free PMC article.
-
NADPH oxidases: molecular understanding finally reaching the clinical level?Antioxid Redox Signal. 2009 Oct;11(10):2365-70. doi: 10.1089/ars.2009.2615. Antioxid Redox Signal. 2009. PMID: 19358633 Free PMC article.
-
Regulation of cytoskeletal dynamics by redox signaling and oxidative stress: implications for neuronal development and trafficking.Front Cell Neurosci. 2015 Sep 30;9:381. doi: 10.3389/fncel.2015.00381. eCollection 2015. Front Cell Neurosci. 2015. PMID: 26483635 Free PMC article. Review.
-
NADPH oxidase in brain injury and neurodegenerative disorders.Mol Neurodegener. 2017 Jan 17;12(1):7. doi: 10.1186/s13024-017-0150-7. Mol Neurodegener. 2017. PMID: 28095923 Free PMC article. Review.
References
-
- Babior, B. M., J. D. Lambeth, and W. Nauseef. 2002. The neutrophil NADPH oxidase. Arch. Biochem. Biophys. 397:342-344. - PubMed
-
- Caston, J., F. Vasseur, T. Stelz, C. Chianale, N. Delhaye-Bouchaud, and J. Mariani. 1995. Differential roles of cerebellar cortex and deep cerebellar nuclei in the learning of the equilibrium behavior: studies in intact and cerebellectomized lurcher mutant mice. Brain Res. Dev. Brain Res. 86:311-316. - PubMed
Publication types
MeSH terms
Substances
Grants and funding
LinkOut - more resources
Full Text Sources
Medical
Molecular Biology Databases