Decreasing pfmdr1 copy number in plasmodium falciparum malaria heightens susceptibility to mefloquine, lumefantrine, halofantrine, quinine, and artemisinin
- PMID: 16845638
- PMCID: PMC2978021
- DOI: 10.1086/507115
Decreasing pfmdr1 copy number in plasmodium falciparum malaria heightens susceptibility to mefloquine, lumefantrine, halofantrine, quinine, and artemisinin
Abstract
The global dissemination of drug-resistant Plasmodium falciparum is spurring intense efforts to implement artemisinin (ART)-based combination therapies for malaria, including mefloquine (MFQ)-artesunate and lumefantrine (LUM)-artemether. Clinical studies have identified an association between an increased risk of MFQ, MFQ-artesunate, and LUM-artemether treatment failures and pfmdr1 gene amplification. To directly address the contribution that pfmdr1 copy number makes to drug resistance, we genetically disrupted 1 of the 2 pfmdr1 copies in the drug-resistant FCB line, which resulted in reduced pfmdr1 mRNA and protein expression. These knockdown clones manifested a 3-fold decrease in MFQ IC(50) values, compared with that for the FCB line, verifying the role played by pfmdr1 expression levels in mediating resistance to MFQ. These clones also showed increased susceptibility to LUM, halofantrine, quinine, and ART. No change was observed for chloroquine. These results highlight the importance of pfmdr1 copy number in determining P. falciparum susceptibility to multiple agents currently being used to combat malaria caused by multidrug-resistant parasites.
Conflict of interest statement
Potential conflicts of interest: none reported.
Figures
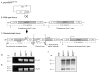
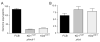
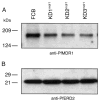
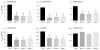
Similar articles
-
Pfmdr1 copy number and arteminisin derivatives combination therapy failure in falciparum malaria in Cambodia.Malar J. 2009 Jan 12;8:11. doi: 10.1186/1475-2875-8-11. Malar J. 2009. PMID: 19138391 Free PMC article.
-
Increased prevalence of the Plasmodium falciparum Pfmdr1 86N genotype among field isolates from Franceville, Gabon after replacement of chloroquine by artemether-lumefantrine and artesunate-mefloquine.Infect Genet Evol. 2011 Mar;11(2):512-7. doi: 10.1016/j.meegid.2011.01.003. Epub 2011 Jan 17. Infect Genet Evol. 2011. PMID: 21251998
-
In vivo selection of Plasmodium falciparum pfmdr1 86N coding alleles by artemether-lumefantrine (Coartem).J Infect Dis. 2005 Mar 15;191(6):1014-7. doi: 10.1086/427997. Epub 2005 Feb 8. J Infect Dis. 2005. PMID: 15717281 Clinical Trial.
-
Contribution of the pfmdr1 gene to antimalarial drug-resistance.Acta Trop. 2005 Jun;94(3):181-90. doi: 10.1016/j.actatropica.2005.04.008. Acta Trop. 2005. PMID: 15876420 Review.
-
The emerging threat of artemisinin resistance in malaria: focus on artemether-lumefantrine.Expert Rev Anti Infect Ther. 2015 Aug;13(8):1031-45. doi: 10.1586/14787210.2015.1052793. Epub 2015 Jun 16. Expert Rev Anti Infect Ther. 2015. PMID: 26081265 Review.
Cited by
-
A novel 4-aminoquinoline chemotype with multistage antimalarial activity and lack of cross-resistance with PfCRT and PfMDR1 mutants.PLoS Pathog. 2024 Oct 29;20(10):e1012627. doi: 10.1371/journal.ppat.1012627. eCollection 2024 Oct. PLoS Pathog. 2024. PMID: 39471233 Free PMC article.
-
In vitro antimalarial susceptibility profile of Plasmodium falciparum isolates in the BEI Resources repository.Antimicrob Agents Chemother. 2024 Oct 8;68(10):e0118923. doi: 10.1128/aac.01189-23. Epub 2024 Sep 13. Antimicrob Agents Chemother. 2024. PMID: 39269188 Free PMC article.
-
Deaggregation of mutant Plasmodium yoelii de-ubiquitinase UBP1 alters MDR1 localization to confer multidrug resistance.Nat Commun. 2024 Feb 27;15(1):1774. doi: 10.1038/s41467-024-46006-3. Nat Commun. 2024. PMID: 38413566 Free PMC article.
-
Emergence, transmission dynamics and mechanisms of artemisinin partial resistance in malaria parasites in Africa.Nat Rev Microbiol. 2024 Jun;22(6):373-384. doi: 10.1038/s41579-024-01008-2. Epub 2024 Feb 6. Nat Rev Microbiol. 2024. PMID: 38321292 Review.
-
Genetic characteristics of P. falciparum parasites collected from 2012 to 2016 and anti-malaria resistance along the China-Myanmar border.PLoS One. 2023 Nov 10;18(11):e0293590. doi: 10.1371/journal.pone.0293590. eCollection 2023. PLoS One. 2023. PMID: 37948402 Free PMC article.
References
-
- Uhlemann AC, Krishna S. Antimalarial multi-drug resistance in Asia: mechanisms and assessment. Curr Top Microbiol Immunol. 2005;295:39–53. - PubMed
-
- Baird JK. Effectiveness of antimalarial drugs. N Engl J Med. 2005;352:1565–77. - PubMed
-
- Wongsrichanalai C, Pickard AL, Wernsdorfer WH, Meshnick SR. Epidemiology of drug-resistant malaria. Lancet Infect Dis. 2002;2:209–18. - PubMed
-
- Uhlemann A-C, Yuthavong Y, Fidock DA. Mechanisms of antimalarial drug action and resistance. In: Sherman I, editor. Molecular approaches to malaria. 2. Washington, DC: ASM Press; 2005. pp. 229–61.
Publication types
MeSH terms
Substances
Grants and funding
LinkOut - more resources
Full Text Sources
Other Literature Sources
Miscellaneous