Venular basement membranes contain specific matrix protein low expression regions that act as exit points for emigrating neutrophils
- PMID: 16754715
- PMCID: PMC2118318
- DOI: 10.1084/jem.20051210
Venular basement membranes contain specific matrix protein low expression regions that act as exit points for emigrating neutrophils
Abstract
The mechanism of leukocyte migration through venular walls in vivo is largely unknown. By using immunofluorescence staining and confocal microscopy, the present study demonstrates the existence of regions within the walls of unstimulated murine cremasteric venules where expression of key vascular basement membrane (BM) constituents, laminin 10, collagen IV, and nidogen-2 (but not perlecan) are considerably lower (<60%) than the average expression detected in the same vessel. These sites were closely associated with gaps between pericytes and were preferentially used by migrating neutrophils during their passage through cytokine-stimulated venules. Although neutrophil transmigration did not alter the number/unit area of extracellular matrix protein low expression sites, the size of these regions was enlarged and their protein content was reduced in interleukin-1beta-stimulated venules. These effects were entirely dependent on the presence of neutrophils and appeared to involve neutrophil-derived serine proteases. Furthermore, evidence was obtained indicating that transmigrating neutrophils carry laminins on their cell surface in vivo. Collectively, through identification of regions of low extracellular matrix protein localization that define the preferred route for transmigrating neutrophils, we have identified a plausible mechanism by which neutrophils penetrate the vascular BM without causing a gross disruption to its intricate structure.
Figures
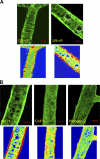
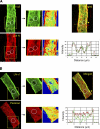
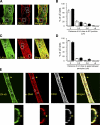
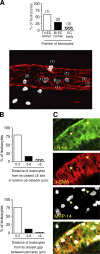
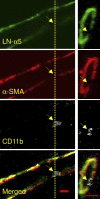
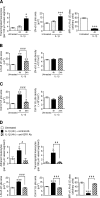
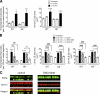
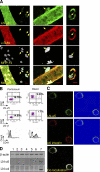
Similar articles
-
Venular basement membranes ubiquitously express matrix protein low-expression regions: characterization in multiple tissues and remodeling during inflammation.Am J Pathol. 2010 Jan;176(1):482-95. doi: 10.2353/ajpath.2010.090510. Epub 2009 Dec 11. Am J Pathol. 2010. PMID: 20008148 Free PMC article.
-
PECAM-1, alpha6 integrins and neutrophil elastase cooperate in mediating neutrophil transmigration.J Cell Sci. 2005 May 1;118(Pt 9):2067-76. doi: 10.1242/jcs.02340. Epub 2005 Apr 19. J Cell Sci. 2005. PMID: 15840647
-
PECAM-1 (CD31) homophilic interaction up-regulates alpha6beta1 on transmigrated neutrophils in vivo and plays a functional role in the ability of alpha6 integrins to mediate leukocyte migration through the perivascular basement membrane.J Exp Med. 2002 Nov 4;196(9):1201-11. doi: 10.1084/jem.20020324. J Exp Med. 2002. PMID: 12417630 Free PMC article.
-
Neutrophil transmigration: emergence of an adhesive cascade within venular walls.J Innate Immun. 2013;5(4):336-47. doi: 10.1159/000346659. Epub 2013 Mar 2. J Innate Immun. 2013. PMID: 23466407 Free PMC article. Review.
-
Transmigration through venular walls: a key regulator of leukocyte phenotype and function.Trends Immunol. 2005 Mar;26(3):157-65. doi: 10.1016/j.it.2005.01.006. Trends Immunol. 2005. PMID: 15745858 Review.
Cited by
-
The extracellular matrix: a dynamic niche in cancer progression.J Cell Biol. 2012 Feb 20;196(4):395-406. doi: 10.1083/jcb.201102147. J Cell Biol. 2012. PMID: 22351925 Free PMC article. Review.
-
The Tumour Vasculature as a Target to Modulate Leucocyte Trafficking.Cancers (Basel). 2021 Apr 6;13(7):1724. doi: 10.3390/cancers13071724. Cancers (Basel). 2021. PMID: 33917287 Free PMC article. Review.
-
Pericytes regulate vascular basement membrane remodeling and govern neutrophil extravasation during inflammation.PLoS One. 2012;7(9):e45499. doi: 10.1371/journal.pone.0045499. Epub 2012 Sep 21. PLoS One. 2012. PMID: 23029055 Free PMC article.
-
Brain mural cell loss in the parietal cortex in Alzheimer's disease correlates with cognitive decline and TDP-43 pathology.Neuropathol Appl Neurobiol. 2020 Aug;46(5):458-477. doi: 10.1111/nan.12599. Epub 2020 Feb 11. Neuropathol Appl Neurobiol. 2020. PMID: 31970820 Free PMC article.
-
Neutrophils recruited by chemoattractants in vivo induce microvascular plasma protein leakage through secretion of TNF.J Exp Med. 2014 Jun 30;211(7):1307-14. doi: 10.1084/jem.20132413. Epub 2014 Jun 9. J Exp Med. 2014. PMID: 24913232 Free PMC article.
References
-
- Vestweber, D., and J.E. Blanks. 1999. Mechanisms that regulate the function of the selectins and their ligands. Physiol. Rev. 79:181–213. - PubMed
-
- Alon, R., and S. Feigelson. 2002. From rolling to arrest on blood vessels: leukocyte tap dancing on endothelial integrin ligands and chemokines at sub-second contacts. Semin. Immunol. 14:93–104. - PubMed
-
- Yadav, R., K.Y. Larbi, R.E. Young, and S. Nourshargh. 2003. Migration of leukocytes through the vessel wall and beyond. Thromb. Haemost. 90:598–606. - PubMed
-
- Mandarino, L.J., N. Sundarraj, J. Finlayson, and J.R. Hassell. 1993. Regulation of fibronectin and laminin synthesis by retinal capillary endothelial cells and pericytes in vitro. Exp. Eye Res. 57:609–621. - PubMed
Publication types
MeSH terms
Substances
Grants and funding
LinkOut - more resources
Full Text Sources
Other Literature Sources
Molecular Biology Databases