Deletion of open reading frame UL26 from the human cytomegalovirus genome results in reduced viral growth, which involves impaired stability of viral particles
- PMID: 16699023
- PMCID: PMC1472153
- DOI: 10.1128/JVI.02585-05
Deletion of open reading frame UL26 from the human cytomegalovirus genome results in reduced viral growth, which involves impaired stability of viral particles
Abstract
We previously showed that open reading frame (ORF) UL26 of human cytomegalovirus, a member of the US22 multigene family of betaherpesviruses, encodes a novel tegument protein, which is imported into cells in the course of viral infection. Moreover, we demonstrated that pUL26 contains a strong transcriptional activation domain and is capable of stimulating the major immediate-early (IE) enhancer-promoter. Since this suggested an important function of pUL26 during the initiation of the viral replicative cycle, we sought to ascertain the relevance of pUL26 by construction of a viral deletion mutant lacking the UL26 ORF using the bacterial artificial chromosome mutagenesis procedure. The resulting deletion virus was verified by PCR, enzyme restriction, and Southern blot analyses. After infection of human foreskin fibroblasts, the UL26 deletion mutant showed a small-plaque phenotype and replicated to significantly lower titers than wild-type or revertant virus. In particular, we noticed a striking decrease of infectious titers 7 days postinfection in a multistep growth experiment, whereas the release of viral DNA from infected cells was not impaired. A further investigation of this aspect revealed a significantly diminished stability of viral particles derived from the UL26 deletion mutant. Consistent with this, we observed that the tegument composition of the deletion mutant deviates from that of the wild-type virus. We therefore hypothesize that pUL26 plays a role not only in the onset of IE gene transcription but also in the assembly of the viral tegument layer in a stable and correct manner.
Figures
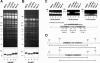
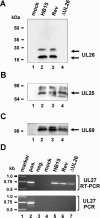
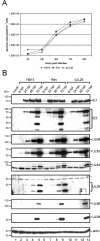
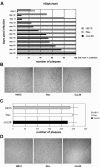
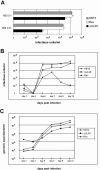
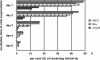
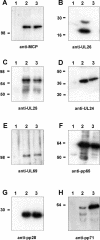
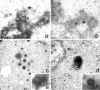
Similar articles
-
Open reading frame UL26 of human cytomegalovirus encodes a novel tegument protein that contains a strong transcriptional activation domain.J Virol. 2002 May;76(10):4836-47. doi: 10.1128/jvi.76.10.4836-4847.2002. J Virol. 2002. PMID: 11967300 Free PMC article.
-
UL26-deficient human cytomegalovirus produces virions with hypophosphorylated pp28 tegument protein that is unstable within newly infected cells.J Virol. 2006 Apr;80(7):3541-8. doi: 10.1128/JVI.80.7.3541-3548.2006. J Virol. 2006. PMID: 16537622 Free PMC article.
-
The Abundant Tegument Protein pUL25 of Human Cytomegalovirus Prevents Proteasomal Degradation of pUL26 and Supports Its Suppression of ISGylation.J Virol. 2018 Nov 27;92(24):e01180-18. doi: 10.1128/JVI.01180-18. Print 2018 Dec 15. J Virol. 2018. PMID: 30282718 Free PMC article.
-
The promoter, transcriptional unit, and coding sequence of herpes simplex virus 1 family 35 proteins are contained within and in frame with the UL26 open reading frame.J Virol. 1991 Jan;65(1):206-12. doi: 10.1128/JVI.65.1.206-212.1991. J Virol. 1991. PMID: 1845885 Free PMC article.
-
Mutant human cytomegalovirus lacking the immediate-early TRS1 coding region exhibits a late defect.J Virol. 2002 Dec;76(23):12290-9. doi: 10.1128/jvi.76.23.12290-12299.2002. J Virol. 2002. PMID: 12414969 Free PMC article.
Cited by
-
Cyclin-Dependent Kinase 8 Represents a Positive Regulator of Cytomegalovirus Replication and a Novel Host Target for Antiviral Strategies.Pharmaceutics. 2024 Sep 23;16(9):1238. doi: 10.3390/pharmaceutics16091238. Pharmaceutics. 2024. PMID: 39339274 Free PMC article.
-
Establishment of a Luciferase-Based Reporter System to Study Aspects of Human Cytomegalovirus Infection, Replication Characteristics, and Antiviral Drug Efficacy.Pathogens. 2024 Jul 31;13(8):645. doi: 10.3390/pathogens13080645. Pathogens. 2024. PMID: 39204245 Free PMC article.
-
Insights into the Transcriptome of Human Cytomegalovirus: A Comprehensive Review.Viruses. 2023 Aug 8;15(8):1703. doi: 10.3390/v15081703. Viruses. 2023. PMID: 37632045 Free PMC article. Review.
-
A Cysteine Residue of Human Cytomegalovirus vMIA Protein Plays a Crucial Role in Viperin Trafficking to Control Viral Infectivity.J Virol. 2023 Jun 29;97(6):e0187422. doi: 10.1128/jvi.01874-22. Epub 2023 Jun 12. J Virol. 2023. PMID: 37306568 Free PMC article.
-
Assessment of Covalently Binding Warhead Compounds in the Validation of the Cytomegalovirus Nuclear Egress Complex as an Antiviral Target.Cells. 2023 Apr 14;12(8):1162. doi: 10.3390/cells12081162. Cells. 2023. PMID: 37190072 Free PMC article.
References
-
- Adler, H., M. Messerle, and U. H. Koszinowski. 2003. Cloning of herpesviral genomes as bacterial artificial chromosomes. Rev. Med. Virol. 13:111-121. - PubMed
-
- Almeida, J., D. Lang, and P. Talbot. 1978. Herpesvirus morphology: visualization of a structural subunit. Intervirology 10:318-320. - PubMed
-
- Andreoni, M., M. Faircloth, L. Vugler, and W. J. Britt. 1989. A rapid microneutralization assay for the measurement of neutralizing antibody reactive with human cytomegalovirus. J. Virol. Methods 23:157-167. - PubMed
Publication types
MeSH terms
Substances
LinkOut - more resources
Full Text Sources
Other Literature Sources