Neocortical network activity in vivo is generated through a dynamic balance of excitation and inhibition
- PMID: 16641233
- PMCID: PMC6674060
- DOI: 10.1523/JNEUROSCI.5297-05.2006
Neocortical network activity in vivo is generated through a dynamic balance of excitation and inhibition
Abstract
The recurrent excitatory and inhibitory connections between and within layers of the cerebral cortex are fundamental to the operation of local cortical circuits. Models of cortical function often assume that recurrent excitation and inhibition are balanced, and we recently demonstrated that spontaneous network activity in vitro contains a precise balance of excitation and inhibition; however, the existence of a balance between excitation and inhibition in the intact and spontaneously active cerebral cortex has not been directly tested. We examined this hypothesis in the prefrontal cortex in vivo, during the slow (<1 Hz) oscillation in ketamine-xylazine-anesthetized ferrets. We measured persistent network activity (Up states) with extracellular multiple unit and local field potential recording, while simultaneously recording synaptic currents in nearby cells. We determined the reversal potential and conductance change over time during Up states and found that the body of Up state activity exhibited a steady reversal potential (-37 mV on average) for hundreds of milliseconds, even during substantial (21 nS on average) changes in membrane conductance. Furthermore, we found that both the initial and final segments of the Up state were characterized by significantly more depolarized reversal potentials and concomitant increases in excitatory conductance, compared with the stable middle portions of Up states. This ongoing temporal evolution between excitation and inhibition, which exhibits remarkable proportionality within and across neurons in active local networks, may allow for rapid transitions between relatively stable network states, permitting the modulation of neuronal responsiveness in a behaviorally relevant manner.
Figures
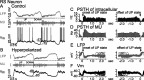
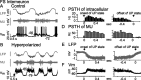
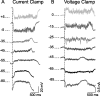
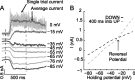
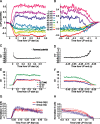
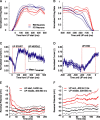
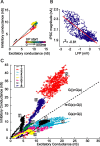
Similar articles
-
Background synaptic activity is sparse in neocortex.J Neurosci. 2006 Aug 9;26(32):8267-77. doi: 10.1523/JNEUROSCI.2152-06.2006. J Neurosci. 2006. PMID: 16899721 Free PMC article.
-
Selective functional interactions between excitatory and inhibitory cortical neurons and differential contribution to persistent activity of the slow oscillation.J Neurosci. 2012 Aug 29;32(35):12165-79. doi: 10.1523/JNEUROSCI.1181-12.2012. J Neurosci. 2012. PMID: 22933799 Free PMC article.
-
Functional consequences of correlated excitatory and inhibitory conductances in cortical networks.J Comput Neurosci. 2010 Jun;28(3):579-94. doi: 10.1007/s10827-010-0240-9. Epub 2010 May 19. J Comput Neurosci. 2010. PMID: 20490645
-
Neuronal circuits of the neocortex.Annu Rev Neurosci. 2004;27:419-51. doi: 10.1146/annurev.neuro.27.070203.144152. Annu Rev Neurosci. 2004. PMID: 15217339 Review.
-
Experimental evidence for sparse firing in the neocortex.Trends Neurosci. 2012 Jun;35(6):345-55. doi: 10.1016/j.tins.2012.03.008. Epub 2012 May 12. Trends Neurosci. 2012. PMID: 22579264 Review.
Cited by
-
Slow dynamics in features of synchronized neural network responses.Front Comput Neurosci. 2015 Apr 14;9:40. doi: 10.3389/fncom.2015.00040. eCollection 2015. Front Comput Neurosci. 2015. PMID: 25926787 Free PMC article.
-
Advantages and limitations of the use of optogenetic approach in studying fast-scale spike encoding.PLoS One. 2015 Apr 7;10(4):e0122286. doi: 10.1371/journal.pone.0122286. eCollection 2015. PLoS One. 2015. PMID: 25850004 Free PMC article.
-
Dynamic interplay of frontoparietal cholinergic innervation and cortical reorganization in the regulation of attentional capacities in aging.Neurobiol Aging. 2021 Sep;105:186-198. doi: 10.1016/j.neurobiolaging.2021.04.027. Epub 2021 May 6. Neurobiol Aging. 2021. PMID: 34102380 Free PMC article.
-
Population-wide distributions of neural activity during perceptual decision-making.Prog Neurobiol. 2013 Apr;103:156-93. doi: 10.1016/j.pneurobio.2012.09.004. Epub 2012 Nov 1. Prog Neurobiol. 2013. PMID: 23123501 Free PMC article. Review.
-
Group I mGluR antagonist rescues the deficit of D1-induced LTP in a mouse model of fragile X syndrome.Mol Neurodegener. 2012 May 28;7:24. doi: 10.1186/1750-1326-7-24. Mol Neurodegener. 2012. PMID: 22640474 Free PMC article.
References
-
- Anderson J, Lampl I, Reichova I, Carandini M, Ferster D (2000). Stimulus dependence of two-state fluctuations of membrane potential in cat visual cortex. Nat Neurosci 3:617–621. - PubMed
-
- Anderson JS, Carandini M, Ferster D (2000). Orientation tuning of input conductance, excitation, and inhibition in cat primary visual cortex. J Neurophysiol 84:909–926. - PubMed
-
- Arieli A, Shoham D, Hildesheim R, Grinvald A (1995). Coherent spatiotemporal patterns of ongoing activity revealed by real-time optical imaging coupled with single-unit recording in the cat visual cortex. J Neurophysiol 73:2072–2093. - PubMed
Publication types
MeSH terms
LinkOut - more resources
Full Text Sources
Miscellaneous