Two-stage mechanism for activation of the DNA replication checkpoint kinase Cds1 in fission yeast
- PMID: 16618806
- PMCID: PMC1472306
- DOI: 10.1101/gad.1406706
Two-stage mechanism for activation of the DNA replication checkpoint kinase Cds1 in fission yeast
Abstract
The DNA replication checkpoint is a complex signal transduction pathway, present in all eukaryotic cells, that functions to maintain genomic integrity and cell viability when DNA replication is perturbed. In Schizosaccharomyces pombe the major effector of the replication checkpoint is the protein kinase Cds1. Activation of Cds1 is known to require the upstream kinase Rad3 and the mediator Mrc1, but the biochemical mechanism of activation is not well understood. We report that the replication checkpoint is activated in two stages. In the first stage, Mrc1 recruits Cds1 to stalled replication forks by interactions between the FHA domain of Cds1 and specific phosphorylated Rad3 consensus sites in Mrc1. Cds1 is then primed for activation by Rad3-dependent phosphorylation. In the second stage, primed Cds1 molecules dimerize via phospho-specific interactions mediated by the FHA domains and are activated by autophosphorylation. This two-stage activation mechanism for the replication checkpoint allows for rapid activation with a high signal-to-noise ratio.
Figures
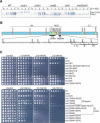
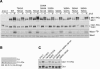
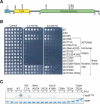
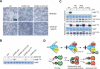
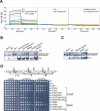
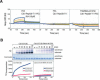
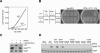
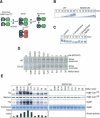
Similar articles
-
Autoinhibition and autoactivation of the DNA replication checkpoint kinase Cds1.J Biol Chem. 2009 Jun 5;284(23):16016-27. doi: 10.1074/jbc.M900785200. Epub 2009 Apr 8. J Biol Chem. 2009. PMID: 19357077 Free PMC article.
-
Replication checkpoint protein Mrc1 is regulated by Rad3 and Tel1 in fission yeast.Mol Cell Biol. 2003 Nov;23(22):8395-403. doi: 10.1128/MCB.23.22.8395-8403.2003. Mol Cell Biol. 2003. PMID: 14585996 Free PMC article.
-
The phosphorylation network for efficient activation of the DNA replication checkpoint in fission yeast.J Biol Chem. 2011 Jul 1;286(26):22864-74. doi: 10.1074/jbc.M111.236687. Epub 2011 May 11. J Biol Chem. 2011. PMID: 21561865 Free PMC article.
-
Cds1 phosphorylation by Rad3-Rad26 kinase is mediated by forkhead-associated domain interaction with Mrc1.J Biol Chem. 2004 Jul 30;279(31):32079-86. doi: 10.1074/jbc.M404834200. Epub 2004 Jun 1. J Biol Chem. 2004. PMID: 15173168
-
Rad3 and Sty1 function in Schizosaccharomyces pombe: an integrated response to DNA damage and environmental stress?Mol Microbiol. 2008 Apr;68(2):246-54. doi: 10.1111/j.1365-2958.2008.06147.x. Mol Microbiol. 2008. PMID: 18366437 Review.
Cited by
-
Autoinhibition and autoactivation of the DNA replication checkpoint kinase Cds1.J Biol Chem. 2009 Jun 5;284(23):16016-27. doi: 10.1074/jbc.M900785200. Epub 2009 Apr 8. J Biol Chem. 2009. PMID: 19357077 Free PMC article.
-
Redundant roles of Srs2 helicase and replication checkpoint in survival and rDNA maintenance in Schizosaccharomyces pombe.Mol Genet Genomics. 2009 May;281(5):497-509. doi: 10.1007/s00438-009-0426-x. Epub 2009 Feb 11. Mol Genet Genomics. 2009. PMID: 19205745
-
Active Replication Checkpoint Drives Genome Instability in Fission Yeast mcm4 Mutant.Mol Cell Biol. 2020 Jun 29;40(14):e00033-20. doi: 10.1128/MCB.00033-20. Print 2020 Jun 29. Mol Cell Biol. 2020. PMID: 32341083 Free PMC article.
-
Checkpoint-Independent Regulation of Origin Firing by Mrc1 through Interaction with Hsk1 Kinase.Mol Cell Biol. 2017 Mar 17;37(7):e00355-16. doi: 10.1128/MCB.00355-16. Print 2017 Apr 1. Mol Cell Biol. 2017. PMID: 28069740 Free PMC article.
-
Mek1 kinase is regulated to suppress double-strand break repair between sister chromatids during budding yeast meiosis.Mol Cell Biol. 2007 Aug;27(15):5456-67. doi: 10.1128/MCB.00416-07. Epub 2007 May 25. Mol Cell Biol. 2007. PMID: 17526735 Free PMC article.
References
-
- Ahn J., Prives C. Checkpoint kinase 2 (Chk2) monomers or dimers phosphorylate Cdc25C after DNA damage regardless of threonine 68 phosphorylation. J. Biol. Chem. 2002;277:48418–48426. - PubMed
-
- Ahn J.Y., Li X., Davis H.L., Canman C.E. Phosphorylation of threonine 68 promotes oligomerization and autophosphorylation of the Chk2 protein kinase via the forkhead-associated domain. J. Biol. Chem. 2002;277:19389–19395. - PubMed
-
- Ahn J., Urist M., Prives C. The Chk2 protein kinase. DNA Repair (Amst.) 2004;3:1039–1047. - PubMed
-
- Alcasabas A.A., Osborn A.J., Bachant J., Hu F., Werler P.J., Bousset K., Furuya K., Diffley J.F., Carr A.M., Elledge S.J. Mrc1 transduces signals of DNA replication stress to activate Rad53. Nat. Cell Biol. 2001;3:958–965. - PubMed
-
- Bartkova J., Horejsi Z., Koed K., Kramer A., Tort F., Zieger K., Guldberg P., Sehested M., Nesland J.M., Lukas C., et al. DNA damage response as a candidate anti-cancer barrier in early human tumorigenesis. Nature. 2005;434:864–870. - PubMed
MeSH terms
Substances
LinkOut - more resources
Full Text Sources
Other Literature Sources
Molecular Biology Databases
Miscellaneous