Reconstitution of the transition from lamellipodium to filopodium in a membrane-free system
- PMID: 16549794
- PMCID: PMC1458768
- DOI: 10.1073/pnas.0508269103
Reconstitution of the transition from lamellipodium to filopodium in a membrane-free system
Abstract
The cellular cytoskeleton is a complex dynamical network that constantly remodels as cells divide and move. This reorganization process occurs not only at the cell membrane, but also in the cell interior (bulk). During locomotion, regulated actin assembly near the plasma membrane produces lamellipodia and filopodia. Therefore, most in vitro experiments explore phenomena taking place in the vicinity of a surface. To understand how the molecular machinery of a cell self-organizes in a more general way, we studied bulk polymerization of actin in the presence of actin-related protein 2/3 complex and a nucleation promoting factor as a model for actin assembly in the cell interior separate from membranes. Bulk polymerization of actin in the presence of the verprolin homology, cofilin homology, and acidic region, domain of Wiskott-Aldrich syndrome protein, and actin-related protein 2/3 complex results in spontaneous formation of diffuse aster-like structures. In the presence of fascin these asters transition into stars with bundles of actin filaments growing from the surface, similar to star-like structures recently observed in vivo. The transition from asters to stars depends on the ratio [fascin]/[G actin]. The polarity of the actin filaments during the transition is preserved, as in the transition from lamellipodia to filopodia. Capping protein inhibits star formation. Based on these experiments and kinetic Monte Carlo simulations, we propose a model for the spontaneous self-assembly of asters and their transition into stars. This mechanism may apply to the transition from lamellipodia to filopodia in vivo.
Conflict of interest statement
Conflict of interest statement: No conflicts declared.
Figures
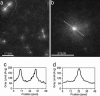
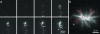
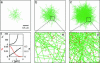
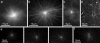
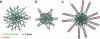
Similar articles
-
Arp2/3 branched actin network mediates filopodia-like bundles formation in vitro.PLoS One. 2008 Sep 29;3(9):e3297. doi: 10.1371/journal.pone.0003297. PLoS One. 2008. PMID: 18820726 Free PMC article.
-
Formation of filopodia-like bundles in vitro from a dendritic network.J Cell Biol. 2003 Mar 17;160(6):951-62. doi: 10.1083/jcb.200208059. J Cell Biol. 2003. PMID: 12642617 Free PMC article.
-
Model of turnover kinetics in the lamellipodium: implications of slow- and fast- diffusing capping protein and Arp2/3 complex.Phys Biol. 2016 Dec 6;13(6):066009. doi: 10.1088/1478-3975/13/6/066009. Phys Biol. 2016. PMID: 27922825 Free PMC article.
-
The making of filopodia.Curr Opin Cell Biol. 2006 Feb;18(1):18-25. doi: 10.1016/j.ceb.2005.11.002. Epub 2005 Dec 6. Curr Opin Cell Biol. 2006. PMID: 16337369 Review.
-
Actin binding proteins: their ups and downs in metastatic life.Cell Adh Migr. 2013 Mar-Apr;7(2):199-213. doi: 10.4161/cam.23176. Epub 2013 Jan 9. Cell Adh Migr. 2013. PMID: 23302954 Free PMC article. Review.
Cited by
-
Arp2/3 branched actin network mediates filopodia-like bundles formation in vitro.PLoS One. 2008 Sep 29;3(9):e3297. doi: 10.1371/journal.pone.0003297. PLoS One. 2008. PMID: 18820726 Free PMC article.
-
Biology under construction: in vitro reconstitution of cellular function.Nat Rev Mol Cell Biol. 2009 Sep;10(9):644-50. doi: 10.1038/nrm2746. Epub 2009 Aug 12. Nat Rev Mol Cell Biol. 2009. PMID: 19672276 Free PMC article. Review.
-
The More the Tubular: Dynamic Bundling of Actin Filaments for Membrane Tube Formation.PLoS Comput Biol. 2016 Jul 6;12(7):e1004982. doi: 10.1371/journal.pcbi.1004982. eCollection 2016 Jul. PLoS Comput Biol. 2016. PMID: 27384915 Free PMC article.
-
Structural, mechanical, and dynamical variability of the actin cortex in living cells.Biophys J. 2015 Mar 24;108(6):1330-1340. doi: 10.1016/j.bpj.2015.01.016. Biophys J. 2015. PMID: 25809247 Free PMC article.
-
Self-organizing actin patterns shape cytoskeletal cortex organization.Commun Integr Biol. 2017 Apr 27;10(3):e1303591. doi: 10.1080/19420889.2017.1303591. eCollection 2017. Commun Integr Biol. 2017. PMID: 28702125 Free PMC article.
References
Publication types
MeSH terms
Substances
Grants and funding
LinkOut - more resources
Full Text Sources