Coordination of microtubule and microfilament dynamics by Drosophila Rho1, Spire and Cappuccino
- PMID: 16518391
- PMCID: PMC1997291
- DOI: 10.1038/ncb1385
Coordination of microtubule and microfilament dynamics by Drosophila Rho1, Spire and Cappuccino
Abstract
The actin-nucleation factors Spire and Cappuccino (Capu) regulate the onset of ooplasmic streaming in Drosophila melanogaster. Although this streaming event is microtubule-based, actin assembly is required for its timing. It is not understood how the interaction of microtubules and microfilaments is mediated in this context. Here, we demonstrate that Capu and Spire have microtubule and microfilament crosslinking activity. The spire locus encodes several distinct protein isoforms (SpireA, SpireC and SpireD). SpireD was recently shown to nucleate actin, but the activity of the other isoforms has not been addressed. We find that SpireD does not have crosslinking activity, whereas SpireC is a potent crosslinker. We show that SpireD binds to Capu and inhibits F-actin/microtubule crosslinking, and activated Rho1 abolishes this inhibition, establishing a mechanistic basis for the regulation of Capu and Spire activity. We propose that Rho1, cappuccino and spire are elements of a conserved developmental cassette that is capable of directly mediating crosstalk between microtubules and microfilaments.
Figures
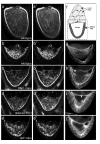
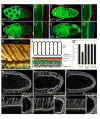
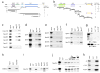
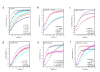
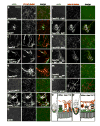
Similar articles
-
Interaction between microtubules and the Drosophila formin Cappuccino and its effect on actin assembly.J Biol Chem. 2014 Feb 14;289(7):4395-404. doi: 10.1074/jbc.M113.499921. Epub 2013 Dec 20. J Biol Chem. 2014. PMID: 24362037 Free PMC article.
-
Regulatory interactions between two actin nucleators, Spire and Cappuccino.J Cell Biol. 2007 Oct 8;179(1):117-28. doi: 10.1083/jcb.200706196. J Cell Biol. 2007. PMID: 17923532 Free PMC article.
-
Capu and Spire assemble a cytoplasmic actin mesh that maintains microtubule organization in the Drosophila oocyte.Dev Cell. 2007 Oct;13(4):539-53. doi: 10.1016/j.devcel.2007.09.003. Dev Cell. 2007. PMID: 17925229 Free PMC article.
-
Cellular functions of the Spir actin-nucleation factors.Trends Cell Biol. 2006 Sep;16(9):477-83. doi: 10.1016/j.tcb.2006.07.005. Epub 2006 Aug 9. Trends Cell Biol. 2006. PMID: 16901698 Review.
-
[Advances in the study of the actin nucleation factor Spire].Sheng Li Xue Bao. 2024 Apr 25;76(2):341-345. Sheng Li Xue Bao. 2024. PMID: 38658382 Review. Chinese.
Cited by
-
Animal Models of Neurodegenerative Disease: Recent Advances in Fly Highlight Innovative Approaches to Drug Discovery.Front Mol Neurosci. 2022 Apr 19;15:883358. doi: 10.3389/fnmol.2022.883358. eCollection 2022. Front Mol Neurosci. 2022. PMID: 35514431 Free PMC article. Review.
-
Analysis tools for single-monomer measurements of self-assembly processes.Sci Rep. 2022 Mar 18;12(1):4682. doi: 10.1038/s41598-022-08245-6. Sci Rep. 2022. PMID: 35304498 Free PMC article.
-
A nucleator arms race: cellular control of actin assembly.Nat Rev Mol Cell Biol. 2010 Apr;11(4):237-51. doi: 10.1038/nrm2867. Epub 2010 Mar 18. Nat Rev Mol Cell Biol. 2010. PMID: 20237478 Free PMC article. Review.
-
Differential interactions of the formins INF2, mDia1, and mDia2 with microtubules.Mol Biol Cell. 2011 Dec;22(23):4575-87. doi: 10.1091/mbc.E11-07-0616. Epub 2011 Oct 12. Mol Biol Cell. 2011. PMID: 21998204 Free PMC article.
-
Formin 2 Regulates Lysosomal Degradation of Wnt-Associated β-Catenin in Neural Progenitors.Cereb Cortex. 2019 May 1;29(5):1938-1952. doi: 10.1093/cercor/bhy073. Cereb Cortex. 2019. PMID: 29659741 Free PMC article.
References
-
- Theurkauf WE. Premature microtubule-dependent cytoplasmic streaming in cappuccino and spire mutant oocytes. Science. 1994;265:2093–2096. - PubMed
-
- Manseau L, Schüpbach T. cappuccino and spire: two unique maternal-effect loci required for both the anteroposterior and dorsoventral patterns of the Drosophila embryo. Genes Dev. 1989;3:1437–1452. - PubMed
-
- Emmons S, et al. cappuccino, a Drosophila maternal effect gene required for polarity of the egg and embryo, is related to the vertebrate limb deformity locus. Genes Dev. 1995;9:2482–2494. - PubMed
-
- Wellington A, et al. Spire contains actin binding domains and is related to ascidian posterior end mark-5. Development. 1999;126:5267–74. - PubMed
-
- Cooley L, Theurkauf WE. Cytoskeletal functions during Drosophila oogenesis. Science. 1994;266:590–6. - PubMed
Publication types
MeSH terms
Substances
Grants and funding
LinkOut - more resources
Full Text Sources
Other Literature Sources
Molecular Biology Databases