Synaptic vesicle protein 2 enhances release probability at quiescent synapses
- PMID: 16436618
- PMCID: PMC6674579
- DOI: 10.1523/JNEUROSCI.2699-05.2006
Synaptic vesicle protein 2 enhances release probability at quiescent synapses
Abstract
We report a thorough analysis of neurotransmission in cultured hippocampal neurons lacking synaptic vesicle protein 2 (SV2), a membrane glycoprotein present in all vesicles that undergo regulated secretion. We found that SV2 selectively enhances low-frequency neurotransmission by priming morphologically docked vesicles. Loss of SV2 reduced initial release probability during a train of action potentials but had no effect on steady-state responses. The amount and decay rate of asynchronous release, two measures sensitive to presynaptic calcium concentrations, are not altered in SV2 knock-outs, suggesting that SV2 does not act by modulating presynaptic calcium. Normal neurotransmission could be temporarily recovered by delivering an exhaustive stimulus train. Our results indicate that SV2 primes vesicles in quiescent neurons and that SV2 function can be bypassed by an activity-dependent priming mechanism. We propose that SV2 action modulates synaptic networks by ensuring that low-frequency neurotransmission is faithfully conveyed.
Figures
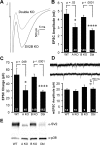
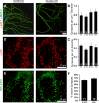
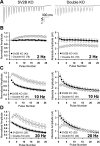
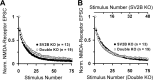
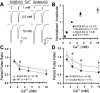
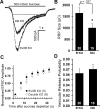
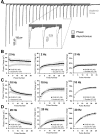
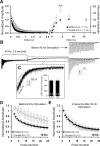
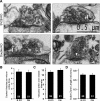
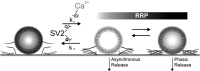
Similar articles
-
The Synaptic Vesicle Glycoprotein 2: Structure, Function, and Disease Relevance.ACS Chem Neurosci. 2019 Sep 18;10(9):3927-3938. doi: 10.1021/acschemneuro.9b00351. Epub 2019 Aug 23. ACS Chem Neurosci. 2019. PMID: 31394034 Free PMC article. Review.
-
SV2 regulates neurotransmitter release via multiple mechanisms.Am J Physiol Cell Physiol. 2010 Nov;299(5):C960-7. doi: 10.1152/ajpcell.00259.2010. Epub 2010 Aug 11. Am J Physiol Cell Physiol. 2010. PMID: 20702688 Free PMC article.
-
SV2 renders primed synaptic vesicles competent for Ca2+ -induced exocytosis.J Neurosci. 2009 Jan 28;29(4):883-97. doi: 10.1523/JNEUROSCI.4521-08.2009. J Neurosci. 2009. PMID: 19176798 Free PMC article.
-
Synaptotagmin I synchronizes transmitter release in mouse hippocampal neurons.J Neurosci. 2004 Jul 7;24(27):6127-32. doi: 10.1523/JNEUROSCI.1563-04.2004. J Neurosci. 2004. PMID: 15240804 Free PMC article.
-
The synaptic vesicle cycle revisited.Neuron. 2000 Nov;28(2):317-20. doi: 10.1016/s0896-6273(00)00109-4. Neuron. 2000. PMID: 11144340 Review. No abstract available.
Cited by
-
Evaluating the In Vivo Specificity of [18F]UCB-H for the SV2A Protein, Compared with SV2B and SV2C in Rats Using microPET.Molecules. 2019 May 1;24(9):1705. doi: 10.3390/molecules24091705. Molecules. 2019. PMID: 31052478 Free PMC article.
-
Synaptic dysfunction induced by glycine-alanine dipeptides in C9orf72-ALS/FTD is rescued by SV2 replenishment.EMBO Mol Med. 2020 May 8;12(5):e10722. doi: 10.15252/emmm.201910722. Epub 2020 Apr 29. EMBO Mol Med. 2020. PMID: 32347002 Free PMC article.
-
SV2B defines a subpopulation of synaptic vesicles.J Mol Cell Biol. 2024 Apr 4;15(9):mjad054. doi: 10.1093/jmcb/mjad054. J Mol Cell Biol. 2024. PMID: 37682518 Free PMC article.
-
Synaptic vesicle protein 2 binds adenine nucleotides.J Biol Chem. 2008 Jul 25;283(30):20628-34. doi: 10.1074/jbc.M800738200. Epub 2008 Jun 4. J Biol Chem. 2008. PMID: 18524768 Free PMC article.
-
The Synaptic Vesicle Glycoprotein 2: Structure, Function, and Disease Relevance.ACS Chem Neurosci. 2019 Sep 18;10(9):3927-3938. doi: 10.1021/acschemneuro.9b00351. Epub 2019 Aug 23. ACS Chem Neurosci. 2019. PMID: 31394034 Free PMC article. Review.
References
-
- Altrock WD, tom Dieck S, Sokolov M, Meyer AC, Sigler A, Brakebusch C, Fassler R, Richter K, Boeckers TM, Potschka H, Brandt C, Loscher W, Grimberg D, Dresbach T, Hempelmann A, Hassan H, Balschun D, Frey JU, Brandstatter JH, Garner CC (2003). Functional inactivation of a fraction of excitatory synapses in mice deficient for the active zone protein bassoon. Neuron 37:787–800. - PubMed
-
- Augustin I, Rosenmund C, Sudhof TC, Brose N (1999). Munc13–1 is essential for fusion competence of glutamatergic synaptic vesicles. Nature 400:457–461. - PubMed
-
- Bajjalieh S (2001). SNAREs take the stage: a prime time to trigger neurotransmitter secretion. Trends Neurosci 24:678–680. - PubMed
-
- Blatow M, Caputi A, Burnashev N, Monyer H, Rozov A (2003). Ca2+ buffer saturation underlies paired pulse facilitation in calbindin-D28k-containing terminals. Neuron 38:79–88. - PubMed
Publication types
MeSH terms
Substances
Grants and funding
LinkOut - more resources
Full Text Sources
Other Literature Sources
Molecular Biology Databases