A pathway for the biogenesis of trans-acting siRNAs in Arabidopsis
- PMID: 16131612
- PMCID: PMC1221887
- DOI: 10.1101/gad.1352605
A pathway for the biogenesis of trans-acting siRNAs in Arabidopsis
Abstract
The Arabidopsis genes, TAS2 and TAS1a, produce structurally similar noncoding transcripts that are transformed into short (21-nucleotide [nt]) and long (24-nt) siRNAs by RNA silencing pathways. Some of these short siRNAs direct the cleavage of protein-coding transcripts, and thus function as trans-acting siRNAs (ta-siRNAs). Using genetic analysis, we defined the pathway by which ta-siRNAs and other short siRNAs are generated from these loci. This process is initiated by the miR173-directed cleavage of a primary poly(A) transcript. The 3' fragment is then transformed into short siRNAs by the sequential activity of SGS3, RDR6, and DCL4: SGS3 stabilizes the fragment, RDR6 produces a complementary strand, and DCL4 cleaves the resulting double-stranded molecule into short siRNAs, starting at the end with the miR173 cleavage site and proceeding in 21-nt increments from this point. The 5' cleavage fragment is also processed by this pathway, but less efficiently. The DCL3-dependent pathway that generates long siRNAs does not require miRNA-directed cleavage and plays a minor role in the silencing of these loci. Our results define the core components of a post-transcriptional gene silencing pathway in Arabidopsis and reveal some of the features that direct transcripts to this pathway.
Figures
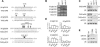
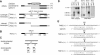
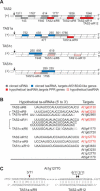
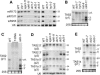
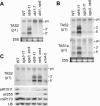
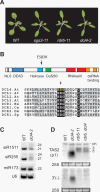
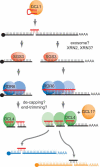
Similar articles
-
Sequencing of RDR6-dependent double-stranded RNAs reveals novel features of plant siRNA biogenesis.Nucleic Acids Res. 2012 Jul;40(13):6241-54. doi: 10.1093/nar/gks242. Epub 2012 Mar 19. Nucleic Acids Res. 2012. PMID: 22434877 Free PMC article.
-
3' fragment of miR173-programmed RISC-cleaved RNA is protected from degradation in a complex with RISC and SGS3.Proc Natl Acad Sci U S A. 2013 Mar 5;110(10):4117-22. doi: 10.1073/pnas.1217050110. Epub 2013 Feb 15. Proc Natl Acad Sci U S A. 2013. PMID: 23417299 Free PMC article.
-
DRB4-dependent TAS3 trans-acting siRNAs control leaf morphology through AGO7.Curr Biol. 2006 May 9;16(9):927-32. doi: 10.1016/j.cub.2006.03.035. Curr Biol. 2006. PMID: 16682354
-
MicroRNA-dependent trans-acting siRNA production.Sci STKE. 2005 Sep 6;2005(300):pe43. doi: 10.1126/stke.3002005pe43. Sci STKE. 2005. PMID: 16145017 Review.
-
The function of RNAi in plant development.Cold Spring Harb Symp Quant Biol. 2006;71:165-70. doi: 10.1101/sqb.2006.71.030. Cold Spring Harb Symp Quant Biol. 2006. PMID: 17381293 Review.
Cited by
-
Roles of dicer-like and argonaute proteins in TAS-derived small interfering RNA-triggered DNA methylation.Plant Physiol. 2012 Oct;160(2):990-9. doi: 10.1104/pp.112.200279. Epub 2012 Jul 30. Plant Physiol. 2012. PMID: 22846193 Free PMC article.
-
Novel DICER-LIKE1 siRNAs Bypass the Requirement for DICER-LIKE4 in Maize Development.Plant Cell. 2015 Aug;27(8):2163-77. doi: 10.1105/tpc.15.00194. Epub 2015 Jul 24. Plant Cell. 2015. PMID: 26209554 Free PMC article.
-
DICER-LIKE2 Plays a Crucial Role in Rice Stripe Virus Coat Protein-Mediated Virus Resistance in Arabidopsis.Viruses. 2023 Nov 10;15(11):2239. doi: 10.3390/v15112239. Viruses. 2023. PMID: 38005916 Free PMC article.
-
Evolutionary patterns and coevolutionary consequences of MIRNA genes and microRNA targets triggered by multiple mechanisms of genomic duplications in soybean.Plant Cell. 2015 Mar;27(3):546-62. doi: 10.1105/tpc.15.00048. Epub 2015 Mar 6. Plant Cell. 2015. PMID: 25747880 Free PMC article.
-
The Rf and Rf-like PPR in higher plants, a fast-evolving subclass of PPR genes.RNA Biol. 2013;10(9):1469-76. doi: 10.4161/rna.25568. Epub 2013 Jul 9. RNA Biol. 2013. PMID: 23872480 Free PMC article. Review.
References
-
- Allen E., Xie, Z., Gustafson, A.M., Sung, G.H., Spatafora, J.W., and Carrington, J.C. 2004. Evolution of microRNA genes by inverted duplication of target gene sequences in Arabidopsis thaliana. Nat. Genet. 36: 1282-1290. - PubMed
-
- Allen E., Xie, Z., Gustafson, A.M., and Carrington, J.C. 2005. microRNA-directed phasing during trans-acting siRNA biogenesis in plants. Cell 121: 207-221. - PubMed
-
- Ambros V. 2004. The functions of animal microRNAs. Nature 431: 350-355. - PubMed
-
- Ambros V., Lee, R.C., Lavanway, A., Williams, P.T., and Jewell, D. 2003. MicroRNAs and other tiny endogenous RNAs in C. elegans. Curr. Biol. 13: 807-818. - PubMed
-
- Aravin A.A., Naumova, N.M., Tulin, A.V., Vagin, V.V., Rozovsky, Y.M., and Gvozdev, V.A. 2001. Double-stranded RNA-mediated silencing of genomic tandem repeats and transposable elements in the D. melanogaster germline. Curr. Biol. 11: 1017-1027. - PubMed
Publication types
MeSH terms
Substances
Grants and funding
LinkOut - more resources
Full Text Sources
Other Literature Sources
Molecular Biology Databases
Research Materials