Depalmitoylated Ras traffics to and from the Golgi complex via a nonvesicular pathway
- PMID: 16027222
- PMCID: PMC2171405
- DOI: 10.1083/jcb.200502063
Depalmitoylated Ras traffics to and from the Golgi complex via a nonvesicular pathway
Abstract
Palmitoylation is postulated to regulate Ras signaling by modulating its intracellular trafficking and membrane microenvironment. The mechanisms by which palmitoylation contributes to these events are poorly understood. Here, we show that dynamic turnover of palmitate regulates the intracellular trafficking of HRas and NRas to and from the Golgi complex by shifting the protein between vesicular and nonvesicular modes of transport. A combination of time-lapse microscopy and photobleaching techniques reveal that in the absence of palmitoylation, GFP-tagged HRas and NRas undergo rapid exchange between the cytosol and ER/Golgi membranes, and that wild-type GFP-HRas and GFP-NRas are recycled to the Golgi complex by a nonvesicular mechanism. Our findings support a model where palmitoylation kinetically traps Ras on membranes, enabling the protein to undergo vesicular transport. We propose that a cycle of depalmitoylation and repalmitoylation regulates the time course and sites of Ras signaling by allowing the protein to be released from the cell surface and rapidly redistributed to intracellular membranes.
Figures
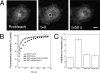
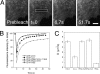
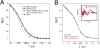
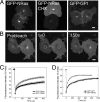
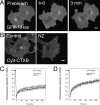
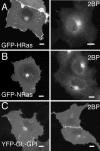
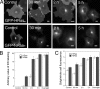
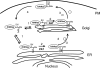
Similar articles
-
A palmitoylation cycle dynamically regulates partitioning of the GABA-synthesizing enzyme GAD65 between ER-Golgi and post-Golgi membranes.J Cell Sci. 2008 Feb 15;121(Pt 4):437-49. doi: 10.1242/jcs.011916. Epub 2008 Jan 29. J Cell Sci. 2008. PMID: 18230651
-
Photobleaching approaches to investigate diffusional mobility and trafficking of Ras in living cells.Methods. 2005 Oct;37(2):154-64. doi: 10.1016/j.ymeth.2005.05.013. Methods. 2005. PMID: 16288889
-
GBF1, a cis-Golgi and VTCs-localized ARF-GEF, is implicated in ER-to-Golgi protein traffic.J Cell Sci. 2006 Sep 15;119(Pt 18):3743-53. doi: 10.1242/jcs.03173. Epub 2006 Aug 22. J Cell Sci. 2006. PMID: 16926190
-
Palmitoylation cycles and regulation of protein function (Review).Mol Membr Biol. 2009 Jan;26(1):42-54. doi: 10.1080/09687680802680108. Epub 2009 Jan 30. Mol Membr Biol. 2009. PMID: 19169934 Review.
-
Maintenance of Golgi apparatus structure in the face of continuous protein recycling to the endoplasmic reticulum: making ends meet.Int Rev Cytol. 2005;244:69-94. doi: 10.1016/S0074-7696(05)44002-4. Int Rev Cytol. 2005. PMID: 16157178 Review.
Cited by
-
VPS35 binds farnesylated N-Ras in the cytosol to regulate N-Ras trafficking.J Cell Biol. 2016 Aug 15;214(4):445-58. doi: 10.1083/jcb.201604061. Epub 2016 Aug 8. J Cell Biol. 2016. PMID: 27502489 Free PMC article.
-
A quantitative approach to analyze binding diffusion kinetics by confocal FRAP.Biophys J. 2010 Nov 3;99(9):2737-47. doi: 10.1016/j.bpj.2010.09.013. Biophys J. 2010. PMID: 21044570 Free PMC article.
-
Clathrin-independent endocytosis: a unique platform for cell signaling and PM remodeling.Cell Signal. 2009 Jan;21(1):1-6. doi: 10.1016/j.cellsig.2008.06.020. Epub 2008 Jul 3. Cell Signal. 2009. PMID: 18647649 Free PMC article. Review.
-
2-Bromopalmitate reduces protein deacylation by inhibition of acyl-protein thioesterase enzymatic activities.PLoS One. 2013 Oct 2;8(10):e75232. doi: 10.1371/journal.pone.0075232. eCollection 2013. PLoS One. 2013. PMID: 24098372 Free PMC article.
-
Palmitoyl protein thioesterase-1 deficiency impairs synaptic vesicle recycling at nerve terminals, contributing to neuropathology in humans and mice.J Clin Invest. 2008 Sep;118(9):3075-86. doi: 10.1172/JCI33482. J Clin Invest. 2008. PMID: 18704195 Free PMC article.
References
-
- Baker, T.L., M.A. Booden, and J.E. Buss. 2000. S-nitrosocysteine increases palmitate turnover on Ha-Ras in NIH 3T3 cells. J. Biol. Chem. 275:22037–22047. - PubMed
-
- Baker, T.L., H. Zheng, J. Walker, J.L. Coloff, and J.E. Buss. 2003. Distinct rates of palmitate turnover on membrane-bound cellular and oncogenic H-ras. J. Biol. Chem. 278:19292–19300. - PubMed
-
- Berthiaume, L., and M.D. Resh. 1995. Biochemical characterization of a palmitoyl acyltransferase activity that palmitoylates myristoylated proteins. J. Biol. Chem. 270:22399–22405. - PubMed
-
- Bivona, T.G., and M.R. Philips. 2003. Ras pathway signaling on endomembranes. Curr. Opin. Cell Biol. 15:136–142. - PubMed
Publication types
MeSH terms
Substances
LinkOut - more resources
Full Text Sources
Research Materials
Miscellaneous