Individual palmitoyl residues serve distinct roles in H-ras trafficking, microlocalization, and signaling
- PMID: 16024806
- PMCID: PMC1190337
- DOI: 10.1128/MCB.25.15.6722-6733.2005
Individual palmitoyl residues serve distinct roles in H-ras trafficking, microlocalization, and signaling
Abstract
H-ras is anchored to the plasma membrane by two palmitoylated cysteine residues, Cys181 and Cys184, operating in concert with a C-terminal S-farnesyl cysteine carboxymethylester. Here we demonstrate that the two palmitates serve distinct biological roles. Monopalmitoylation of Cys181 is required and sufficient for efficient trafficking of H-ras to the plasma membrane, whereas monopalmitoylation of Cys184 does not permit efficient trafficking beyond the Golgi apparatus. However, once at the plasma membrane, monopalmitoylation of Cys184 supports correct GTP-regulated lateral segregation of H-ras between cholesterol-dependent and cholesterol-independent microdomains. In contrast, monopalmitoylation of Cys181 dramatically reverses H-ras lateral segregation, driving GTP-loaded H-ras into cholesterol-dependent microdomains. Intriguingly, the Cys181 monopalmitoylated H-ras anchor emulates the GTP-regulated microdomain interactions of N-ras. These results identify N-ras as the Ras isoform that normally signals from lipid rafts but also reveal that spacing between palmitate and prenyl groups influences anchor interactions with the lipid bilayer. This concept is further supported by the different plasma membrane affinities of the monopalmitoylated anchors: Cys181-palmitate is equivalent to the dually palmitoylated wild-type anchor, whereas Cys184-palmitate is weaker. Thus, membrane affinity of a palmitoylated anchor is a function both of the hydrophobicity of the lipid moieties and their spatial organization. Finally we show that the plasma membrane affinity of monopalmitoylated anchors is absolutely dependent on cholesterol, identifying a new role for cholesterol in promoting interactions with the raft and nonraft plasma membrane.
Figures
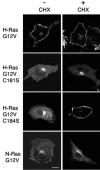
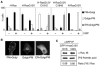
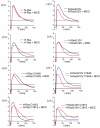
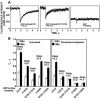
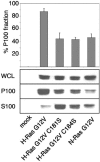
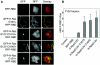
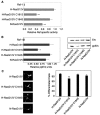
Similar articles
-
Similar membrane affinity of mono- and Di-S-acylated ras membrane anchors: a new twist in the role of protein lipidation.J Am Chem Soc. 2008 Sep 24;130(38):12624-5. doi: 10.1021/ja805110q. Epub 2008 Aug 30. J Am Chem Soc. 2008. PMID: 18761454 Free PMC article.
-
Three separable domains regulate GTP-dependent association of H-ras with the plasma membrane.Mol Cell Biol. 2004 Aug;24(15):6799-810. doi: 10.1128/MCB.24.15.6799-6810.2004. Mol Cell Biol. 2004. PMID: 15254246 Free PMC article.
-
Transient palmitoylation supports H-Ras membrane binding but only partial biological activity.Biochemistry. 1999 Sep 28;38(39):12926-34. doi: 10.1021/bi9909290. Biochemistry. 1999. PMID: 10504264
-
FRAP beam-size analysis to measure palmitoylation-dependent membrane association dynamics and microdomain partitioning of Ras proteins.Methods. 2006 Oct;40(2):183-90. doi: 10.1016/j.ymeth.2006.02.003. Methods. 2006. PMID: 17012031 Review.
-
Nonconventional trafficking of Ras associated with Ras signal organization.Traffic. 2006 Sep;7(9):119-26. doi: 10.1111/j.1600-0854.2006.00459.x. Traffic. 2006. PMID: 16824054 Review.
Cited by
-
Phosphatidylinositol-3,4,5-trisphosphate: tool of choice for class I PI 3-kinases.Bioessays. 2013 Jul;35(7):602-11. doi: 10.1002/bies.201200176. Bioessays. 2013. PMID: 23765576 Free PMC article. Review.
-
Plasma membrane localization of Ras requires class C Vps proteins and functional mitochondria in Saccharomyces cerevisiae.Mol Cell Biol. 2006 Apr;26(8):3243-55. doi: 10.1128/MCB.26.8.3243-3255.2006. Mol Cell Biol. 2006. PMID: 16581797 Free PMC article.
-
Caveolae regulate the nanoscale organization of the plasma membrane to remotely control Ras signaling.J Cell Biol. 2014 Mar 3;204(5):777-92. doi: 10.1083/jcb.201307055. Epub 2014 Feb 24. J Cell Biol. 2014. PMID: 24567358 Free PMC article.
-
Raft protein clustering alters N-Ras membrane interactions and activation pattern.Mol Cell Biol. 2011 Oct;31(19):3938-52. doi: 10.1128/MCB.05570-11. Epub 2011 Aug 1. Mol Cell Biol. 2011. PMID: 21807892 Free PMC article.
-
Acylation of CD44 and its association with lipid rafts are required for receptor and hyaluronan endocytosis.J Biol Chem. 2006 Nov 10;281(45):34601-9. doi: 10.1074/jbc.M601530200. Epub 2006 Aug 30. J Biol Chem. 2006. PMID: 16945930 Free PMC article.
References
-
- Baker, T. L., H. Zheng, J. Walker, J. L. Coloff, and J. E. Buss. 2003. Distinct rates of palmitate turnover on membrane-bound cellular and oncogenic H-ras. J. Biol. Chem. 278:19292-19300. - PubMed
-
- Besag, J. E. 1977. Contribution to the discussion of Dr. Ripley's paper. J. R. Statist. Soc. B. 39:193-195.
-
- Bivona, T. G., I. Perez De Castro, I. M. Ahearn, T. M. Grana, V. K. Chiu, P. J. Lockyer, P. J. Cullen, A. Pellicer, A. D. Cox, and M. R. Philips. 2003. Phospholipase Cgamma activates Ras on the Golgi apparatus apparatus by means of RasGRP1. Nature 424:694-698. - PubMed
Publication types
MeSH terms
Substances
Grants and funding
LinkOut - more resources
Full Text Sources
Research Materials
Miscellaneous