The role of heterochromatin in centromere function
- PMID: 15905142
- PMCID: PMC1569473
- DOI: 10.1098/rstb.2004.1611
The role of heterochromatin in centromere function
Abstract
Chromatin at centromeres is distinct from the chromatin in which the remainder of the genome is assembled. Two features consistently distinguish centromeres: the presence of the histone H3 variant CENP-A and, in most organisms, the presence of heterochromatin. In fission yeast, domains of silent "heterochromatin" flank the CENP-A chromatin domain that forms a platform upon which the kinetochore is assembled. Thus, fission yeast centromeres resemble their metazoan counterparts where the kinetochore is embedded in centromeric heterochromatin. The centromeric outer repeat chromatin is underacetylated on histones H3 and H4, and methylated on lysine 9 of histone H3, which provides a binding site for the chromodomain protein Swi6 (orthologue of Heterochromatin Protein 1, HP1). The remarkable demonstration that the assembly of repressive heterochromatin is dependent on the RNA interference machinery provokes many questions about the mechanisms of this process that may be tractable in fission yeast. Heterochromatin ensures that a high density of cohesin is recruited to centromeric regions, but it could have additional roles in centromere architecture and the prevention of merotely, and it might also act as a trigger for kinetochore assembly. In addition, we discuss an epigenetic model for ensuring that CENP-A is targeted and replenished at the kinetochore domain.
Figures
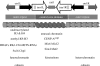
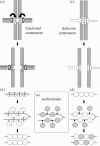
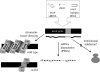
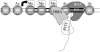
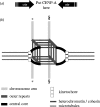
Similar articles
-
Kinetochore and heterochromatin domains of the fission yeast centromere.Chromosome Res. 2004;12(6):521-34. doi: 10.1023/B:CHRO.0000036586.81775.8b. Chromosome Res. 2004. PMID: 15289660 Review.
-
cis-acting DNA from fission yeast centromeres mediates histone H3 methylation and recruitment of silencing factors and cohesin to an ectopic site.Curr Biol. 2002 Oct 1;12(19):1652-60. doi: 10.1016/s0960-9822(02)01177-6. Curr Biol. 2002. PMID: 12361567
-
How to build a centromere: from centromeric and pericentromeric chromatin to kinetochore assembly.Biochem Cell Biol. 2006 Aug;84(4):619-39. doi: 10.1139/o06-078. Biochem Cell Biol. 2006. PMID: 16936833 Review.
-
Heterochromatin and RNAi are required to establish CENP-A chromatin at centromeres.Science. 2008 Jan 4;319(5859):94-7. doi: 10.1126/science.1150944. Science. 2008. PMID: 18174443 Free PMC article.
-
Heterochromatin tells CENP-A where to go.Bioessays. 2008 Jun;30(6):526-9. doi: 10.1002/bies.20763. Bioessays. 2008. PMID: 18478529 Review.
Cited by
-
Molecular analysis of core kinetochore composition and assembly in Drosophila melanogaster.PLoS One. 2007 May 30;2(5):e478. doi: 10.1371/journal.pone.0000478. PLoS One. 2007. PMID: 17534428 Free PMC article.
-
Multivalent engagement of chromatin modifications by linked binding modules.Nat Rev Mol Cell Biol. 2007 Dec;8(12):983-94. doi: 10.1038/nrm2298. Nat Rev Mol Cell Biol. 2007. PMID: 18037899 Free PMC article. Review.
-
Rad51 suppresses gross chromosomal rearrangement at centromere in Schizosaccharomyces pombe.EMBO J. 2008 Nov 19;27(22):3036-46. doi: 10.1038/emboj.2008.215. Epub 2008 Oct 16. EMBO J. 2008. PMID: 18923422 Free PMC article.
-
CFDP1 regulates the stability of pericentric heterochromatin thereby affecting RAN GTPase activity and mitotic spindle formation.PLoS Biol. 2024 Apr 17;22(4):e3002574. doi: 10.1371/journal.pbio.3002574. eCollection 2024 Apr. PLoS Biol. 2024. PMID: 38630655 Free PMC article.
-
Epigenetic Regulation of Chromatin States in Schizosaccharomyces pombe.Cold Spring Harb Perspect Biol. 2015 Jul 1;7(7):a018770. doi: 10.1101/cshperspect.a018770. Cold Spring Harb Perspect Biol. 2015. PMID: 26134317 Free PMC article. Review.
References
-
- Allshire R.C, Javerzat J.P, Redhead N.J, Cranston G. Position effect variegation at fission yeast centromeres. Cell. 1994;76:157–169. - PubMed
-
- Allshire R.C, Nimmo E.R, Ekwall K, Javerzat J.P, Cranston G. Mutations derepressing silent centromeric domains in fission yeast disrupt chromosome segregation. Genes Dev. 1995;9:218–233. - PubMed
Publication types
MeSH terms
Substances
LinkOut - more resources
Full Text Sources
Research Materials