Mycobacterium tuberculosis isocitrate lyases 1 and 2 are jointly required for in vivo growth and virulence
- PMID: 15895072
- PMCID: PMC1464426
- DOI: 10.1038/nm1252
Mycobacterium tuberculosis isocitrate lyases 1 and 2 are jointly required for in vivo growth and virulence
Abstract
Genes involved in fatty acid catabolism have undergone extensive duplication in the genus Mycobacterium, which includes the etiologic agents of leprosy and tuberculosis. Here, we show that prokaryotic- and eukaryotic-like isoforms of the glyoxylate cycle enzyme isocitrate lyase (ICL) are jointly required for fatty acid catabolism and virulence in Mycobacterium tuberculosis. Although deletion of icl1 or icl2, the genes that encode ICL1 and ICL2, respectively, had little effect on bacterial growth in macrophages and mice, deletion of both genes resulted in complete impairment of intracellular replication and rapid elimination from the lungs. The feasibility of targeting ICL1 and ICL2 for chemical inhibition was shown using a dual-specific ICL inhibitor, which blocked growth of M. tuberculosis on fatty acids and in macrophages. The absence of ICL orthologs in mammals should facilitate the development of glyoxylate cycle inhibitors as new drugs for the treatment of tuberculosis.
Conflict of interest statement
COMPETING INTERESTS STATEMENT
The authors declare that they have no competing financial interests.
Figures
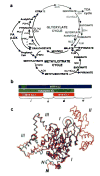
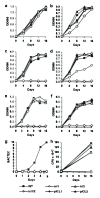
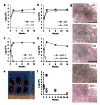
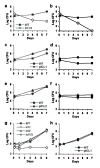
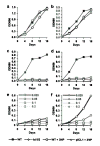
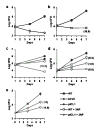
Comment in
-
A low-carb diet for a high-octane pathogen.Nat Med. 2005 Jun;11(6):599-600. doi: 10.1038/nm0605-599. Nat Med. 2005. PMID: 15937469 No abstract available.
Similar articles
-
Role of the methylcitrate cycle in Mycobacterium tuberculosis metabolism, intracellular growth, and virulence.Mol Microbiol. 2006 Jun;60(5):1109-22. doi: 10.1111/j.1365-2958.2006.05155.x. Mol Microbiol. 2006. PMID: 16689789
-
Role of the methylcitrate cycle in propionate metabolism and detoxification in Mycobacterium smegmatis.Microbiology (Reading). 2007 Dec;153(Pt 12):3973-3982. doi: 10.1099/mic.0.2007/011726-0. Microbiology (Reading). 2007. PMID: 18048912
-
Identification of a novel inhibitor of isocitrate lyase as a potent antitubercular agent against both active and non-replicating Mycobacterium tuberculosis.Tuberculosis (Edinb). 2016 Mar;97:38-46. doi: 10.1016/j.tube.2015.12.003. Epub 2016 Jan 6. Tuberculosis (Edinb). 2016. PMID: 26980494
-
Potential inhibitors for isocitrate lyase of Mycobacterium tuberculosis and non-M. tuberculosis: a summary.Biomed Res Int. 2015;2015:895453. doi: 10.1155/2015/895453. Epub 2015 Jan 8. Biomed Res Int. 2015. PMID: 25649791 Free PMC article. Review.
-
Lessons Learnt and the Way Forward for Drug Development Against Isocitrate Lyase from Mycobacterium tuberculosis.Protein Pept Lett. 2022;29(12):1031-1041. doi: 10.2174/0929866529666221006121831. Protein Pept Lett. 2022. PMID: 36201276 Review.
Cited by
-
Characterization of a Functional Role of the Bradyrhizobium japonicum Isocitrate Lyase in Desiccation Tolerance.Int J Mol Sci. 2015 Jul 22;16(7):16695-709. doi: 10.3390/ijms160716695. Int J Mol Sci. 2015. PMID: 26204840 Free PMC article.
-
Itaconate is a covalent inhibitor of the Mycobacterium tuberculosis isocitrate lyase.RSC Med Chem. 2020 Oct 19;12(1):57-61. doi: 10.1039/d0md00301h. eCollection 2021 Jan 1. RSC Med Chem. 2020. PMID: 34046597 Free PMC article.
-
Preferential use of central metabolism in vivo reveals a nutritional basis for polymicrobial infection.PLoS Pathog. 2015 Jan 8;11(1):e1004601. doi: 10.1371/journal.ppat.1004601. eCollection 2015 Jan. PLoS Pathog. 2015. PMID: 25568946 Free PMC article.
-
The Sculpting of the Mycobacterium tuberculosis Genome by Host Cell-Derived Pressures.Microbiol Spectr. 2014 Oct;2(5):10.1128/microbiolspec.MGM2-0016-2013. doi: 10.1128/microbiolspec.MGM2-0016-2013. Microbiol Spectr. 2014. PMID: 25584198 Free PMC article. Review.
-
Regulatory role of Mycobacterium tuberculosis MtrA on dormancy/resuscitation revealed by a novel target gene-mining strategy.Front Microbiol. 2024 Jun 17;15:1415554. doi: 10.3389/fmicb.2024.1415554. eCollection 2024. Front Microbiol. 2024. PMID: 38952446 Free PMC article.
References
-
- Charpentier E, Tuomanen E. Mechanisms of antibiotic resistance and tolerance in Streptococcus pneumoniae. Microbes Infect. 2000;2:1855–1864. - PubMed
-
- Gomez JE, McKinney JD. Mycobacterium tuberculosis persistence, latency, and drug tolerance. Tuberculosis. 2004;84:29–44. - PubMed
-
- Boshoff HI, Barry CE., 3rd Tuberculosis - metabolism and respiration in the absence of growth. Nat Rev Microbiol. 2005;3:70–80. - PubMed
Publication types
MeSH terms
Substances
Grants and funding
LinkOut - more resources
Full Text Sources
Other Literature Sources