Long-range RNA-RNA interactions circularize the dengue virus genome
- PMID: 15890901
- PMCID: PMC1112138
- DOI: 10.1128/JVI.79.11.6631-6643.2005
Long-range RNA-RNA interactions circularize the dengue virus genome
Abstract
Secondary and tertiary RNA structures present in viral RNA genomes play essential regulatory roles during translation, RNA replication, and assembly of new viral particles. In the case of flaviviruses, RNA-RNA interactions between the 5' and 3' ends of the genome have been proposed to be required for RNA replication. We found that two RNA elements present at the ends of the dengue virus genome interact in vitro with high affinity. Visualization of individual molecules by atomic force microscopy revealed that physical interaction between these RNA elements results in cyclization of the viral RNA. Using RNA binding assays, we found that the putative cyclization sequences, known as 5' and 3' CS, present in all mosquito-borne flaviviruses, were necessary but not sufficient for RNA-RNA interaction. Additional sequences present at the 5' and 3' untranslated regions of the viral RNA were also required for RNA-RNA complex formation. We named these sequences 5' and 3' UAR (upstream AUG region). In order to investigate the functional role of 5'-3' UAR complementarity, these sequences were mutated either separately, to destroy base pairing, or simultaneously, to restore complementarity in the context of full-length dengue virus RNA. Nonviable viruses were recovered after transfection of dengue virus RNA carrying mutations either at the 5' or 3' UAR, while the RNA containing the compensatory mutations was able to replicate. Since sequence complementarity between the ends of the genome is required for dengue virus viability, we propose that cyclization of the RNA is a required conformation for viral replication.
Figures
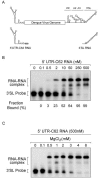
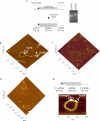
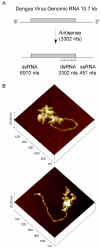
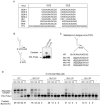
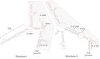
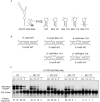
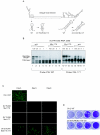
Similar articles
-
Functional analysis of dengue virus cyclization sequences located at the 5' and 3'UTRs.Virology. 2008 May 25;375(1):223-35. doi: 10.1016/j.virol.2008.01.014. Epub 2008 Mar 4. Virology. 2008. PMID: 18289628
-
Interplay of RNA elements in the dengue virus 5' and 3' ends required for viral RNA replication.J Virol. 2010 Jun;84(12):6103-18. doi: 10.1128/JVI.02042-09. Epub 2010 Mar 31. J Virol. 2010. PMID: 20357095 Free PMC article.
-
Structural and functional analysis of dengue virus RNA.Novartis Found Symp. 2006;277:120-32; discussion 132-5, 251-3. Novartis Found Symp. 2006. PMID: 17319158 Review.
-
Essential role of cyclization sequences in flavivirus RNA replication.J Virol. 2001 Jul;75(14):6719-28. doi: 10.1128/JVI.75.14.6719-6728.2001. J Virol. 2001. PMID: 11413342 Free PMC article.
-
Dynamic RNA structures in the dengue virus genome.RNA Biol. 2011 Mar-Apr;8(2):249-57. doi: 10.4161/rna.8.2.14992. Epub 2011 Mar 1. RNA Biol. 2011. PMID: 21593583 Review.
Cited by
-
An RNA element at the 5'-end of the poliovirus genome functions as a general promoter for RNA synthesis.PLoS Pathog. 2010 Jun 3;6(6):e1000936. doi: 10.1371/journal.ppat.1000936. PLoS Pathog. 2010. PMID: 20532207 Free PMC article.
-
Conformational organization of the 3' untranslated region in the tomato bushy stunt virus genome.RNA. 2006 Dec;12(12):2199-210. doi: 10.1261/rna.238606. Epub 2006 Oct 31. RNA. 2006. PMID: 17077273 Free PMC article.
-
Different Degrees of 5'-to-3' DAR Interactions Modulate Zika Virus Genome Cyclization and Host-Specific Replication.J Virol. 2020 Feb 14;94(5):e01602-19. doi: 10.1128/JVI.01602-19. Print 2020 Feb 14. J Virol. 2020. PMID: 31826997 Free PMC article.
-
The Multiples Fates of the Flavivirus RNA Genome During Pathogenesis.Front Genet. 2018 Dec 4;9:595. doi: 10.3389/fgene.2018.00595. eCollection 2018. Front Genet. 2018. PMID: 30564270 Free PMC article. Review.
-
An RNA-binding protein, hnRNP A1, and a scaffold protein, septin 6, facilitate hepatitis C virus replication.J Virol. 2007 Apr;81(8):3852-65. doi: 10.1128/JVI.01311-06. Epub 2007 Jan 17. J Virol. 2007. PMID: 17229681 Free PMC article.
References
-
- Andersen, E. S., S. A. Contera, B. Knudsen, C. K. Damgaard, F. Besenbacher, and J. Kjems. 2004. Role of the trans-activation response element in dimerization of HIV-1 RNA. J. Biol. Chem. 279:22243-22249. - PubMed
-
- Andreev, I. A., S. H. Kim, N. O. Kalinina, D. V. Rakitina, A. G. Fitzgerald, P. Palukaitis, and M. E. Taliansky. 2004. Molecular interactions between a plant virus movement protein and RNA: force spectroscopy investigation. J. Mol. Biol. 339:1041-1047. - PubMed
-
- Barends, S., H. H. Bink, S. H. van den Worm, C. W. Pleij, and B. Kraal. 2003. Entrapping ribosomes for viral translation: tRNA mimicry as a molecular Trojan horse. Cell 112:123-129. - PubMed
Publication types
MeSH terms
Substances
LinkOut - more resources
Full Text Sources
Other Literature Sources