Hsp70 chaperones: cellular functions and molecular mechanism
- PMID: 15770419
- PMCID: PMC2773841
- DOI: 10.1007/s00018-004-4464-6
Hsp70 chaperones: cellular functions and molecular mechanism
Abstract
Hsp70 proteins are central components of the cellular network of molecular chaperones and folding catalysts. They assist a large variety of protein folding processes in the cell by transient association of their substrate binding domain with short hydrophobic peptide segments within their substrate proteins. The substrate binding and release cycle is driven by the switching of Hsp70 between the low-affinity ATP bound state and the high-affinity ADP bound state. Thus, ATP binding and hydrolysis are essential in vitro and in vivo for the chaperone activity of Hsp70 proteins. This ATPase cycle is controlled by co-chaperones of the family of J-domain proteins, which target Hsp70s to their substrates, and by nucleotide exchange factors, which determine the lifetime of the Hsp70-substrate complex. Additional co-chaperones fine-tune this chaperone cycle. For specific tasks the Hsp70 cycle is coupled to the action of other chaperones, such as Hsp90 and Hsp100.
Figures
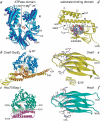
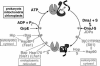
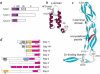
Similar articles
-
Human Hsp70 molecular chaperone binds two calcium ions within the ATPase domain.Structure. 1997 Mar 15;5(3):403-14. doi: 10.1016/s0969-2126(97)00197-4. Structure. 1997. PMID: 9083109
-
Multistep mechanism of substrate binding determines chaperone activity of Hsp70.Nat Struct Biol. 2000 Jul;7(7):586-93. doi: 10.1038/76819. Nat Struct Biol. 2000. PMID: 10876246
-
Investigation of the interaction between DnaK and DnaJ by surface plasmon resonance spectroscopy.J Mol Biol. 1999 Jun 18;289(4):1131-44. doi: 10.1006/jmbi.1999.2844. J Mol Biol. 1999. PMID: 10369787
-
Nucleotide Exchange Factors for Hsp70 Molecular Chaperones: GrpE, Hsp110/Grp170, HspBP1/Sil1, and BAG Domain Proteins.Subcell Biochem. 2023;101:1-39. doi: 10.1007/978-3-031-14740-1_1. Subcell Biochem. 2023. PMID: 36520302 Review.
-
The Hsp70 chaperone network.Nat Rev Mol Cell Biol. 2019 Nov;20(11):665-680. doi: 10.1038/s41580-019-0133-3. Nat Rev Mol Cell Biol. 2019. PMID: 31253954 Review.
Cited by
-
Induction of endoplasmic reticulum stress genes, BiP and chop, in genetic and environmental models of retinal degeneration.Invest Ophthalmol Vis Sci. 2012 Nov 9;53(12):7590-9. doi: 10.1167/iovs.12-10221. Invest Ophthalmol Vis Sci. 2012. PMID: 23074209 Free PMC article.
-
Strain Variation Can Significantly Modulate the miRNA Response to Zika Virus Infection.Int J Mol Sci. 2023 Nov 11;24(22):16216. doi: 10.3390/ijms242216216. Int J Mol Sci. 2023. PMID: 38003407 Free PMC article.
-
Modulation of the chaperone DnaK allosterism by the nucleotide exchange factor GrpE.J Biol Chem. 2015 Apr 17;290(16):10083-92. doi: 10.1074/jbc.M114.623371. Epub 2015 Mar 4. J Biol Chem. 2015. PMID: 25739641 Free PMC article.
-
Gene expression and morphological responses of Lolium perenne L. exposed to cadmium (Cd2+) and mercury (Hg2+).Sci Rep. 2021 May 27;11(1):11257. doi: 10.1038/s41598-021-90826-y. Sci Rep. 2021. PMID: 34045631 Free PMC article.
-
An interdomain energetic tug-of-war creates the allosterically active state in Hsp70 molecular chaperones.Cell. 2012 Dec 7;151(6):1296-307. doi: 10.1016/j.cell.2012.11.002. Cell. 2012. PMID: 23217711 Free PMC article.
References
-
- {'text': '', 'ref_index': 1, 'ids': [{'type': 'PubMed', 'value': '10786831', 'is_inner': True, 'url': 'https://pubmed.ncbi.nlm.nih.gov/10786831/'}]}
- Bukau B., Deuerling E., Pfund C. and Craig E. A. (2000) Getting newly synthesized proteins into shape. Cell 101: 119–122 - PubMed
-
- {'text': '', 'ref_index': 1, 'ids': [{'type': 'PubMed', 'value': '11884745', 'is_inner': True, 'url': 'https://pubmed.ncbi.nlm.nih.gov/11884745/'}]}
- Hartl F. U. and Hayer-Hartl M. (2002) Molecular chaperones in the cytosol: from nascent chain to folded protein. Science 295: 1852–1858. - PubMed
-
- {'text': '', 'ref_index': 1, 'ids': [{'type': 'PubMed', 'value': '14559183', 'is_inner': True, 'url': 'https://pubmed.ncbi.nlm.nih.gov/14559183/'}]}
- Young J. C., Barral J. M. and Ulrich Hartl F. (2003) More than folding: localized functions of cytosolic chaperones. Trends. Biochem. Sci. 28: 541–547 - PubMed
-
- {'text': '', 'ref_index': 1, 'ids': [{'type': 'PubMed', 'value': '12154367', 'is_inner': True, 'url': 'https://pubmed.ncbi.nlm.nih.gov/12154367/'}]}
- Neupert W. and Brunner M. (2002) The protein import motor of mitochondria. Nat. Rev. Mol. Cell. Biol. 3: 555–565 - PubMed
-
- {'text': '', 'ref_index': 1, 'ids': [{'type': 'PubMed', 'value': '11868273', 'is_inner': True, 'url': 'https://pubmed.ncbi.nlm.nih.gov/11868273/'}]}
- Ryan M. T. and Pfanner N. (2002) Hsp70 proteins in protein translocation. Adv. Protein Chem. 59: 223–242 - PubMed
Publication types
MeSH terms
Substances
LinkOut - more resources
Full Text Sources
Other Literature Sources
Molecular Biology Databases