Compartment-resolved imaging of activity-dependent dynamics of cortical blood volume and oximetry
- PMID: 15745949
- PMCID: PMC6726087
- DOI: 10.1523/JNEUROSCI.3032-04.2005
Compartment-resolved imaging of activity-dependent dynamics of cortical blood volume and oximetry
Abstract
Optical imaging, positron emission tomography, and functional magnetic resonance imaging (fMRI) all rely on vascular responses to image neuronal activity. Although these imaging techniques are used successfully for functional brain mapping, the detailed spatiotemporal dynamics of hemodynamic events in the various microvascular compartments have remained unknown. Here we used high-resolution optical imaging in area 18 of anesthetized cats to selectively explore sensory-evoked cerebral blood-volume (CBV) changes in the various cortical microvascular compartments. To avoid the confounding effects of hematocrit and oximetry changes, we developed and used a new fluorescent blood plasma tracer and combined these measurements with optical imaging of intrinsic signals at a near-isosbestic wavelength for hemoglobin (565 nm). The vascular response began at the arteriolar level, rapidly spreading toward capillaries and venules. Larger veins lagged behind. Capillaries exhibited clear blood-volume changes. Arterioles and arteries had the largest response, whereas the venous response was smallest. Information about compartment-specific oxygen tension dynamics was obtained in imaging sessions using 605 nm illumination, a wavelength known to reflect primarily oximetric changes, thus being more directly related to electrical activity than CBV changes. Those images were radically different: the response began at the parenchyma level, followed only later by the other microvascular compartments. These results have implications for the modeling of fMRI responses (e.g., the balloon model). Furthermore, functional maps obtained by imaging the capillary CBV response were similar but not identical to those obtained using the early oximetric signal, suggesting the presence of different regulatory mechanisms underlying these two hemodynamic processes.
Figures
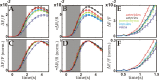
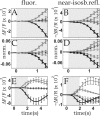
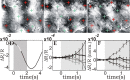
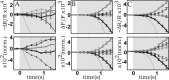
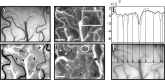
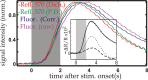
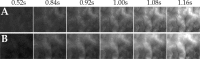
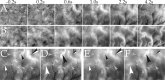
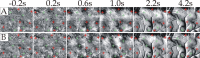
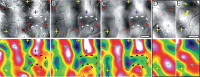
Similar articles
-
Mapping iso-orientation columns by contrast agent-enhanced functional magnetic resonance imaging: reproducibility, specificity, and evaluation by optical imaging of intrinsic signal.J Neurosci. 2006 Nov 15;26(46):11821-32. doi: 10.1523/JNEUROSCI.3098-06.2006. J Neurosci. 2006. PMID: 17108155 Free PMC article.
-
Columnar resolution of blood volume and oximetry functional maps in the behaving monkey; implications for FMRI.Neuron. 2004 Jun 10;42(5):843-54. doi: 10.1016/j.neuron.2004.04.004. Neuron. 2004. PMID: 15182722
-
A processing work-flow for measuring erythrocytes velocity in extended vascular networks from wide field high-resolution optical imaging data.Neuroimage. 2012 Feb 1;59(3):2569-88. doi: 10.1016/j.neuroimage.2011.08.081. Epub 2011 Sep 8. Neuroimage. 2012. PMID: 21925275
-
In Vivo Observations of Rapid Scattered Light Changes Associated with Neurophysiological Activity.In: Frostig RD, editor. In Vivo Optical Imaging of Brain Function. 2nd edition. Boca Raton (FL): CRC Press/Taylor & Francis; 2009. Chapter 5. In: Frostig RD, editor. In Vivo Optical Imaging of Brain Function. 2nd edition. Boca Raton (FL): CRC Press/Taylor & Francis; 2009. Chapter 5. PMID: 26844322 Free Books & Documents. Review.
-
Linking brain vascular physiology to hemodynamic response in ultra-high field MRI.Neuroimage. 2018 Mar;168:279-295. doi: 10.1016/j.neuroimage.2017.02.063. Epub 2017 Feb 22. Neuroimage. 2018. PMID: 28254456 Review.
Cited by
-
Temporal profiles and 2-dimensional oxy-, deoxy-, and total-hemoglobin somatosensory maps in rat versus mouse cortex.Neuroimage. 2007;37 Suppl 1(Suppl 1):S27-36. doi: 10.1016/j.neuroimage.2007.04.063. Epub 2007 May 21. Neuroimage. 2007. PMID: 17574868 Free PMC article.
-
Mapping iso-orientation columns by contrast agent-enhanced functional magnetic resonance imaging: reproducibility, specificity, and evaluation by optical imaging of intrinsic signal.J Neurosci. 2006 Nov 15;26(46):11821-32. doi: 10.1523/JNEUROSCI.3098-06.2006. J Neurosci. 2006. PMID: 17108155 Free PMC article.
-
Regulation of blood flow in the retinal trilaminar vascular network.J Neurosci. 2014 Aug 20;34(34):11504-13. doi: 10.1523/JNEUROSCI.1971-14.2014. J Neurosci. 2014. PMID: 25143628 Free PMC article.
-
MRI contrast agents for functional molecular imaging of brain activity.Curr Opin Neurobiol. 2007 Oct;17(5):593-600. doi: 10.1016/j.conb.2007.11.002. Curr Opin Neurobiol. 2007. PMID: 18093824 Free PMC article. Review.
-
Cellular and physiological mechanisms underlying blood flow regulation in the retina and choroid in health and disease.Prog Retin Eye Res. 2012 Sep;31(5):377-406. doi: 10.1016/j.preteyeres.2012.04.004. Epub 2012 May 3. Prog Retin Eye Res. 2012. PMID: 22580107 Free PMC article. Review.
References
-
- Ances BM, Buerk DG, Greenberg JH, Detre JA (2001) Temporal dynamics of the partial pressure of brain tissue oxygen during functional forepaw stimulation in rats. Neurosci Lett 306: 106-110. - PubMed
-
- Bonhoeffer T, Grinvald A (1991) Iso-orientation domains in cat visual cortex are arranged in pinwheel-like patterns. Nature 353: 429-431. - PubMed
-
- Buxton RB, Frank LR (1997) A model for the coupling between cerebral blood flow and oxygen metabolism during neural stimulation. J Cereb Blood Flow Metab 17: 64-72. - PubMed
Publication types
MeSH terms
Substances
LinkOut - more resources
Full Text Sources
Other Literature Sources
Medical
Miscellaneous