Normal gating of CFTR requires ATP binding to both nucleotide-binding domains and hydrolysis at the second nucleotide-binding domain
- PMID: 15623556
- PMCID: PMC544308
- DOI: 10.1073/pnas.0408575102
Normal gating of CFTR requires ATP binding to both nucleotide-binding domains and hydrolysis at the second nucleotide-binding domain
Abstract
ATP interacts with the two nucleotide-binding domains (NBDs) of CFTR to control gating. However, it is unclear whether gating involves ATP binding alone, or also involves hydrolysis at each NBD. We introduced phenylalanine residues into nonconserved positions of each NBD Walker A motif to sterically prevent ATP binding. These mutations blocked [alpha-(32)P]8-N(3)-ATP labeling of the mutated NBD and reduced channel opening rate without changing burst duration. Introducing cysteine residues at these positions and modifying with N-ethylmaleimide produced the same gating behavior. These results indicate that normal gating requires ATP binding to both NBDs, but ATP interaction with one NBD is sufficient to support some activity. We also studied mutations of the conserved Walker A lysine residues (K464A and K1250A) that prevent hydrolysis. By combining substitutions that block ATP binding with Walker A lysine mutations, we could differentiate the role of ATP binding vs. hydrolysis at each NBD. The K1250A mutation prolonged burst duration; however, blocking ATP binding prevented the long bursts. These data indicate that ATP binding to NBD2 allowed channel opening and that closing was delayed in the absence of hydrolysis. The corresponding NBD1 mutations showed relatively little effect of preventing ATP hydrolysis but a large inhibition of blocking ATP binding. These data suggest that ATP binding to NBD1 is required for normal activity but that hydrolysis has little effect. Our results suggest that both NBDs contribute to channel gating, NBD1 binds ATP but supports little hydrolysis, and ATP binding and hydrolysis at NBD2 are key for normal gating.
Figures
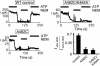
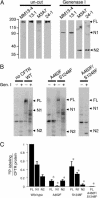
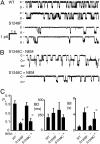
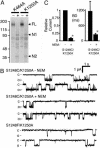
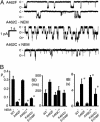
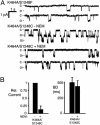
Similar articles
-
On the mechanism of MgATP-dependent gating of CFTR Cl- channels.J Gen Physiol. 2003 Jan;121(1):17-36. doi: 10.1085/jgp.20028673. J Gen Physiol. 2003. PMID: 12508051 Free PMC article.
-
The two ATP binding sites of cystic fibrosis transmembrane conductance regulator (CFTR) play distinct roles in gating kinetics and energetics.J Gen Physiol. 2006 Oct;128(4):413-22. doi: 10.1085/jgp.200609622. Epub 2006 Sep 11. J Gen Physiol. 2006. PMID: 16966475 Free PMC article.
-
The two nucleotide-binding domains of cystic fibrosis transmembrane conductance regulator (CFTR) have distinct functions in controlling channel activity.J Biol Chem. 1995 Jan 27;270(4):1711-7. doi: 10.1074/jbc.270.4.1711. J Biol Chem. 1995. PMID: 7530246
-
Review. ATP hydrolysis-driven gating in cystic fibrosis transmembrane conductance regulator.Philos Trans R Soc Lond B Biol Sci. 2009 Jan 27;364(1514):247-55. doi: 10.1098/rstb.2008.0191. Philos Trans R Soc Lond B Biol Sci. 2009. PMID: 18957373 Free PMC article. Review.
-
Gating of the CFTR Cl- channel by ATP-driven nucleotide-binding domain dimerisation.J Physiol. 2009 May 15;587(Pt 10):2151-61. doi: 10.1113/jphysiol.2009.171595. Epub 2009 Mar 30. J Physiol. 2009. PMID: 19332488 Free PMC article. Review.
Cited by
-
Converting nonhydrolyzable nucleotides to strong cystic fibrosis transmembrane conductance regulator (CFTR) agonists by gain of function (GOF) mutations.J Biol Chem. 2013 Jun 14;288(24):17122-33. doi: 10.1074/jbc.M112.442582. Epub 2013 Apr 25. J Biol Chem. 2013. PMID: 23620589 Free PMC article.
-
Mutating the Conserved Q-loop Glutamine 1291 Selectively Disrupts Adenylate Kinase-dependent Channel Gating of the ATP-binding Cassette (ABC) Adenylate Kinase Cystic Fibrosis Transmembrane Conductance Regulator (CFTR) and Reduces Channel Function in Primary Human Airway Epithelia.J Biol Chem. 2015 May 29;290(22):14140-53. doi: 10.1074/jbc.M114.611616. Epub 2015 Apr 17. J Biol Chem. 2015. PMID: 25887396 Free PMC article.
-
Portrait of multifaceted transporter, the multidrug resistance-associated protein 1 (MRP1/ABCC1).Pflugers Arch. 2007 Feb;453(5):621-41. doi: 10.1007/s00424-006-0160-8. Epub 2006 Dec 23. Pflugers Arch. 2007. PMID: 17187268 Review.
-
The H-loop in the second nucleotide-binding domain of the cystic fibrosis transmembrane conductance regulator is required for efficient chloride channel closing.Cell Physiol Biochem. 2010;25(2-3):169-80. doi: 10.1159/000276549. Epub 2010 Jan 12. Cell Physiol Biochem. 2010. PMID: 20110677 Free PMC article.
-
Sequences in the nonconsensus nucleotide-binding domain of ABCG5/ABCG8 required for sterol transport.J Biol Chem. 2011 Mar 4;286(9):7308-14. doi: 10.1074/jbc.M110.210880. Epub 2011 Jan 5. J Biol Chem. 2011. PMID: 21209088 Free PMC article.
References
-
- Riordan, J. R., Rommens, J. M., Kerem, B. S., Alon, N., Rozmahel, R., Grzelczak, Z., Zielenski, J., Lok, S., Plavsic, N., Chou, J. L., et al. (1989) Science 245, 1066-1073. - PubMed
-
- Welsh, M. J., Ramsey, B. W., Accurso, F. & Cutting, G. R. (2001) in The Metabolic and Molecular Basis of Inherited Disease, eds. Scriver, C. R., Beaudet, A. L., Sly, W. S., Valle, D., Childs, B. & Vogelstein, B. (McGraw–Hill, New York), pp. 5121-5189.
-
- Hung, L. W., Wang, I. X., Nikaido, K., Liu, P. Q., Ames, G. F. L. & Kim, S. H. (1998) Nature 396, 703-707. - PubMed
Publication types
MeSH terms
Substances
Grants and funding
LinkOut - more resources
Full Text Sources