Influence of RNA secondary structure on the pre-mRNA splicing process
- PMID: 15572659
- PMCID: PMC533984
- DOI: 10.1128/MCB.24.24.10505-10514.2004
Influence of RNA secondary structure on the pre-mRNA splicing process
Figures
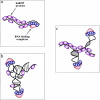
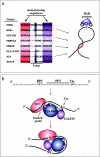
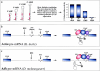
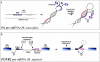
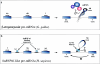
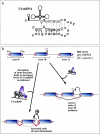
Similar articles
-
Secondary structure of the yeast Saccharomyces cerevisiae pre-U3A snoRNA and its implication for splicing efficiency.RNA. 1996 Nov;2(11):1079-93. RNA. 1996. PMID: 8903339 Free PMC article.
-
Genetics. Minor splicing, disrupted.Science. 2011 Apr 8;332(6026):184-5. doi: 10.1126/science.1205503. Science. 2011. PMID: 21474744 No abstract available.
-
U2 toggles iteratively between the stem IIa and stem IIc conformations to promote pre-mRNA splicing.Genes Dev. 2007 Apr 1;21(7):821-34. doi: 10.1101/gad.1536107. Genes Dev. 2007. PMID: 17403782 Free PMC article.
-
RNA Splicing by the Spliceosome.Annu Rev Biochem. 2020 Jun 20;89:359-388. doi: 10.1146/annurev-biochem-091719-064225. Epub 2019 Dec 3. Annu Rev Biochem. 2020. PMID: 31794245 Review.
-
How Is Precursor Messenger RNA Spliced by the Spliceosome?Annu Rev Biochem. 2020 Jun 20;89:333-358. doi: 10.1146/annurev-biochem-013118-111024. Epub 2019 Dec 9. Annu Rev Biochem. 2020. PMID: 31815536 Review.
Cited by
-
Positional mapping and candidate gene analysis of the mouse Ccs3 locus that regulates differential susceptibility to carcinogen-induced colorectal cancer.PLoS One. 2013;8(3):e58733. doi: 10.1371/journal.pone.0058733. Epub 2013 Mar 14. PLoS One. 2013. PMID: 23516545 Free PMC article.
-
Splicing regulation in spinal muscular atrophy by an RNA structure formed by long-distance interactions.Ann N Y Acad Sci. 2015 Apr;1341:176-87. doi: 10.1111/nyas.12727. Epub 2015 Feb 27. Ann N Y Acad Sci. 2015. PMID: 25727246 Free PMC article.
-
Conserved long-range base pairings are associated with pre-mRNA processing of human genes.Nat Commun. 2021 Apr 16;12(1):2300. doi: 10.1038/s41467-021-22549-7. Nat Commun. 2021. PMID: 33863890 Free PMC article.
-
EDA Fibronectin in Keloids Create a Vicious Cycle of Fibrotic Tumor Formation.J Invest Dermatol. 2015 Jul;135(7):1714-1718. doi: 10.1038/jid.2015.155. J Invest Dermatol. 2015. PMID: 26066891
-
Pyrvinium pamoate changes alternative splicing of the serotonin receptor 2C by influencing its RNA structure.Nucleic Acids Res. 2013 Apr 1;41(6):3819-32. doi: 10.1093/nar/gkt063. Epub 2013 Feb 7. Nucleic Acids Res. 2013. PMID: 23393189 Free PMC article.
References
-
- Adams, M. D., D. Z. Rudner, and D. C. Rio. 1996. Biochemistry and regulation of pre-mRNA splicing. Curr. Opin. Cell Biol. 8:331-339. - PubMed
-
- Antson, A. A. 2000. Single-stranded-RNA binding proteins. Curr. Opin. Struct. Biol. 10:87-94. - PubMed
-
- Balvay, L., D. Libri, and M. Y. Fiszman. 1993. Pre-mRNA secondary structure and the regulation of splicing. Bioessays 15:165-169. - PubMed
-
- Black, D. L. 1991. Does steric interference between splice sites block the splicing of a short c-src neuron-specific exon in non-neuronal cells? Genes Dev. 5:389-402. - PubMed
Publication types
MeSH terms
Substances
LinkOut - more resources
Full Text Sources
Other Literature Sources