Evidence that translocation of anthrax toxin's lethal factor is initiated by entry of its N terminus into the protective antigen channel
- PMID: 15548616
- PMCID: PMC534726
- DOI: 10.1073/pnas.0405754101
Evidence that translocation of anthrax toxin's lethal factor is initiated by entry of its N terminus into the protective antigen channel
Abstract
Entry of the enzymatic components of anthrax toxin [lethal factor (LF) and edema factor] into the cytosol of mammalian cells depends on the ability of the activated protective antigen (PA63) component to form a channel (pore) in the membrane of an acidic intracellular compartment. To investigate the mechanism of translocation, we characterized N-terminally truncated forms of the PA63-binding domain of LF (LFN). Deleting 27 or 36 residues strongly inhibited acid-triggered translocation of LFN across the plasma membrane of CHO-K1 cells and ablated the protein's ability to block PA63 channels in planar lipid bilayers at a small positive voltage (+20 mV). Fusing a H6-tag to the N terminus of the truncated proteins restored both translocation and channel-blocking activities. At +20 mV, N-terminal H6 and biotin tags were accessible to Ni2+ and streptavidin, respectively, added to the trans compartment of a planar bilayer. On the basis of these findings, we propose that the N terminus of PA63-bound LF or edema factor enters the PA63-channel under the influence of acidic pH and a positive transmembrane potential and initiates translocation in an N- to C-terminal direction.
Figures
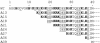
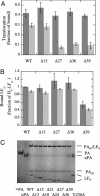
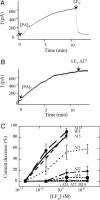
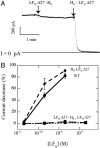
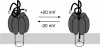
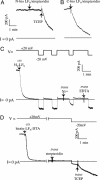
Similar articles
-
Characterization of membrane translocation by anthrax protective antigen.Biochemistry. 1998 Nov 10;37(45):15737-46. doi: 10.1021/bi981436i. Biochemistry. 1998. PMID: 9843379
-
Protein translocation through anthrax toxin channels formed in planar lipid bilayers.Biophys J. 2004 Dec;87(6):3842-9. doi: 10.1529/biophysj.104.050864. Epub 2004 Sep 17. Biophys J. 2004. PMID: 15377524 Free PMC article.
-
Effect of anthrax toxin's lethal factor on ion channels formed by the protective antigen.J Biol Chem. 1995 Aug 4;270(31):18626-30. doi: 10.1074/jbc.270.31.18626. J Biol Chem. 1995. PMID: 7543106
-
The channel formed in planar lipid bilayers by the protective antigen component of anthrax toxin.Toxicology. 1994 Feb 28;87(1-3):29-41. doi: 10.1016/0300-483x(94)90153-8. Toxicology. 1994. PMID: 7512762 Review.
-
Anthrax toxin channel: What we know based on over 30 years of research.Biochim Biophys Acta Biomembr. 2021 Nov 1;1863(11):183715. doi: 10.1016/j.bbamem.2021.183715. Epub 2021 Jul 30. Biochim Biophys Acta Biomembr. 2021. PMID: 34332985 Review.
Cited by
-
Solution Structures of Bacillus anthracis Protective Antigen Proteins Using Small Angle Neutron Scattering and Protective Antigen 63 Ion Channel Formation Kinetics.Toxins (Basel). 2021 Dec 11;13(12):888. doi: 10.3390/toxins13120888. Toxins (Basel). 2021. PMID: 34941724 Free PMC article.
-
Inhibition of anthrax lethal toxin-induced cytolysis of RAW264.7 cells by celastrol.PLoS One. 2008 Jan 9;3(1):e1421. doi: 10.1371/journal.pone.0001421. PLoS One. 2008. PMID: 18183301 Free PMC article.
-
Trapping a translocating protein within the anthrax toxin channel: implications for the secondary structure of permeating proteins.J Gen Physiol. 2011 Apr;137(4):343-56. doi: 10.1085/jgp.201010578. Epub 2011 Mar 14. J Gen Physiol. 2011. PMID: 21402886 Free PMC article.
-
Solubilization and characterization of the anthrax toxin pore in detergent micelles.Protein Sci. 2009 Sep;18(9):1882-95. doi: 10.1002/pro.199. Protein Sci. 2009. PMID: 19609933 Free PMC article.
-
A conserved motif in transmembrane helix 1 of diphtheria toxin mediates catalytic domain delivery to the cytosol.Proc Natl Acad Sci U S A. 2005 Oct 25;102(43):15635-40. doi: 10.1073/pnas.0504937102. Epub 2005 Oct 17. Proc Natl Acad Sci U S A. 2005. PMID: 16230620 Free PMC article.
References
-
- Collier, R. J. & Young, J. A. (2003) Annu. Rev. Cell Dev. Biol. 19, 45–70. - PubMed
-
- Leppla, S. H. (2000) in Bacterial Protein Toxins, eds. Aktories, K. & Just, I. (Springer, Berlin), pp. 445–472.
-
- Pimental, R. A., Christensen, K. A., Krantz, B. A. & Collier, R. J. (2004) Biochem. Biophys. Res. Commun. 322, 258–262. - PubMed
-
- Milne, J. C., Furlong, D., Hanna, P. C., Wall, J. S. & Collier, R. J. (1994) J. Biol. Chem. 269, 20607–20612. - PubMed
Publication types
MeSH terms
Substances
Grants and funding
LinkOut - more resources
Full Text Sources
Other Literature Sources