Mutations that rescue the paralysis of Caenorhabditis elegans ric-8 (synembryn) mutants activate the G alpha(s) pathway and define a third major branch of the synaptic signaling network
- PMID: 15489510
- PMCID: PMC1449092
- DOI: 10.1534/genetics.104.032334
Mutations that rescue the paralysis of Caenorhabditis elegans ric-8 (synembryn) mutants activate the G alpha(s) pathway and define a third major branch of the synaptic signaling network
Abstract
To identify hypothesized missing components of the synaptic G alpha(o)-G alpha(q) signaling network, which tightly regulates neurotransmitter release, we undertook two large forward genetic screens in the model organism C. elegans and focused first on mutations that strongly rescue the paralysis of ric-8(md303) reduction-of-function mutants, previously shown to be defective in G alpha(q) pathway activation. Through high-resolution mapping followed by sequence analysis, we show that these mutations affect four genes. Two activate the G alpha(q) pathway through gain-of-function mutations in G alpha(q); however, all of the remaining mutations activate components of the G alpha(s) pathway, including G alpha(s), adenylyl cyclase, and protein kinase A. Pharmacological assays suggest that the G alpha(s) pathway-activating mutations increase steady-state neurotransmitter release, and the strongly impaired neurotransmitter release of ric-8(md303) mutants is rescued to greater than wild-type levels by the strongest G alpha(s) pathway activating mutations. Using transgene induction studies, we show that activating the G alpha(s) pathway in adult animals rapidly induces hyperactive locomotion and rapidly rescues the paralysis of the ric-8 mutant. Using cell-specific promoters we show that neuronal, but not muscle, G alpha(s) pathway activation is sufficient to rescue ric-8(md303)'s paralysis. Our results appear to link RIC-8 (synembryn) and a third major G alpha pathway, the G alpha(s) pathway, with the previously discovered G alpha(o) and G alpha(q) pathways of the synaptic signaling network.
Figures
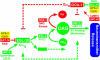
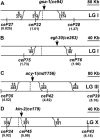
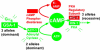
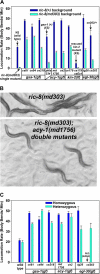
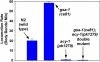
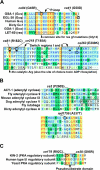
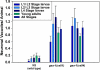
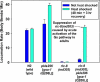
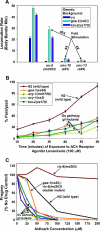
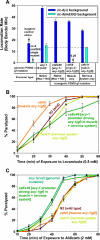
Similar articles
-
Convergent, RIC-8-dependent Galpha signaling pathways in the Caenorhabditis elegans synaptic signaling network.Genetics. 2005 Feb;169(2):651-70. doi: 10.1534/genetics.104.031286. Epub 2004 Oct 16. Genetics. 2005. PMID: 15489511 Free PMC article.
-
RIC-8 (Synembryn): a novel conserved protein that is required for G(q)alpha signaling in the C. elegans nervous system.Neuron. 2000 Aug;27(2):289-99. doi: 10.1016/s0896-6273(00)00037-4. Neuron. 2000. PMID: 10985349 Free PMC article.
-
A role for RIC-8 (Synembryn) and GOA-1 (G(o)alpha) in regulating a subset of centrosome movements during early embryogenesis in Caenorhabditis elegans.Genetics. 2000 Dec;156(4):1649-60. doi: 10.1093/genetics/156.4.1649. Genetics. 2000. PMID: 11102364 Free PMC article.
-
Olfactory G proteins: simple and complex signal transduction.Curr Biol. 1998 Jun 4;8(12):R431-3. doi: 10.1016/s0960-9822(98)70271-4. Curr Biol. 1998. PMID: 9637917 Review.
-
Heterotrimeric G proteins in C. elegans.WormBook. 2006 Oct 13:1-25. doi: 10.1895/wormbook.1.75.1. WormBook. 2006. PMID: 18050432 Free PMC article. Review.
Cited by
-
A longitudinal study of Caenorhabditis elegans larvae reveals a novel locomotion switch, regulated by G(αs) signaling.Elife. 2013 Jul 2;2:e00782. doi: 10.7554/eLife.00782. Elife. 2013. PMID: 23840929 Free PMC article.
-
Novel DLK-independent neuronal regeneration in Caenorhabditis elegans shares links with activity-dependent ectopic outgrowth.Proc Natl Acad Sci U S A. 2016 May 17;113(20):E2852-60. doi: 10.1073/pnas.1600564113. Epub 2016 Apr 12. Proc Natl Acad Sci U S A. 2016. PMID: 27078101 Free PMC article.
-
Dopamine D1- and D2-like receptors oppositely regulate lifespan via a dietary restriction mechanism in Caenorhabditis elegans.BMC Biol. 2022 Mar 23;20(1):71. doi: 10.1186/s12915-022-01272-9. BMC Biol. 2022. PMID: 35317792 Free PMC article.
-
Opposing action of the FLR-2 glycoprotein hormone and DRL-1/FLR-4 MAP kinases balance p38-mediated growth and lipid homeostasis in C. elegans.PLoS Biol. 2023 Sep 29;21(9):e3002320. doi: 10.1371/journal.pbio.3002320. eCollection 2023 Sep. PLoS Biol. 2023. PMID: 37773960 Free PMC article.
-
Neural and molecular dissection of a C. elegans sensory circuit that regulates fat and feeding.Cell Metab. 2008 Aug;8(2):118-31. doi: 10.1016/j.cmet.2008.06.005. Cell Metab. 2008. PMID: 18680713 Free PMC article.
References
-
- Ahnert-Hilger, G., T. Schäfer, K. Spicher, C. Grund, G. Schultz et al., 1994. Detection of G-protein heterotrimers on large dense core and small synaptic vesicles of neuroendocrine and neuronal cells. Eur. J. Cell Biol. 65: 26–38. - PubMed
-
- Aravamudan, B., T. Fergestad, W. S. Davis, C. K. Rodesch and K. Broadie, 1999. Drosophila Unc-13 is essential for synaptic transmission. Nat. Neurosci. 2(11): 965–971. - PubMed
-
- Augustin, I., C. Rosenmund, T. C. Südhof and N. Brose, 1999. Munc13–1 is essential for fusion competence of glutamatergic synaptic vesicles. Nature 400: 457–461. - PubMed
Publication types
MeSH terms
Substances
Grants and funding
LinkOut - more resources
Full Text Sources
Other Literature Sources
Molecular Biology Databases
Research Materials