Tissue plasminogen activator is a potent activator of PDGF-CC
- PMID: 15372073
- PMCID: PMC522796
- DOI: 10.1038/sj.emboj.7600397
Tissue plasminogen activator is a potent activator of PDGF-CC
Abstract
Tissue plasminogen activator (tPA) is a serine protease involved in the degradation of blood clots through the activation of plasminogen to plasmin. Here we report on the identification of tPA as a specific protease able to activate platelet-derived growth factor C (PDGF-C). The newly identified PDGF-C is secreted as a latent dimeric factor (PDGF-CC) that upon proteolytic removal of the N-terminal CUB domains becomes a PDGF receptor alpha agonist. The CUB domains in PDGF-CC directly interact with tPA, and fibroblasts from tPA-deficient mice fail to activate latent PDGF-CC. We further demonstrate that growth of primary fibroblasts in culture is dependent on a tPA-mediated cleavage of latent PDGF-CC, generating a growth stimulatory loop. Immunohistochemical analysis showed similar expression patterns of PDGF-C and tPA in developing mouse embryos and in tumors, indicating both autocrine and paracrine modes of activation of PDGF receptor-mediated signaling pathways. The identification of tPA as an activator of PDGF signaling establishes a novel role for the protease in normal and pathological tissue growth and maintenance, distinct from its well-known role in plasminogen activation and fibrinolysis.
Figures
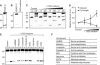
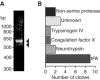
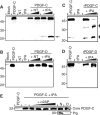
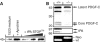
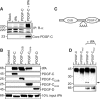
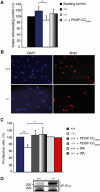
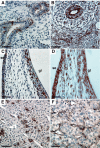
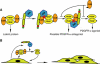
Similar articles
-
Structural requirements for activation of latent platelet-derived growth factor CC by tissue plasminogen activator.J Biol Chem. 2005 Jul 22;280(29):26856-62. doi: 10.1074/jbc.M503388200. Epub 2005 May 23. J Biol Chem. 2005. PMID: 15911618
-
Tissue-type plasminogen activator is not necessary for platelet-derived growth factor-c activation.Biochim Biophys Acta. 2014 Feb;1842(2):318-25. doi: 10.1016/j.bbadis.2013.11.013. Epub 2013 Nov 19. Biochim Biophys Acta. 2014. PMID: 24269585 Free PMC article.
-
Activation of the epidermal growth factor signalling pathway by tissue plasminogen activator in pancreas cancer cells.Gut. 2007 Sep;56(9):1266-74. doi: 10.1136/gut.2006.097188. Epub 2007 Apr 23. Gut. 2007. PMID: 17452424 Free PMC article.
-
[Structure and function of PDGF-R-alpha and its expression in normal kidney and kidney diseases].Przegl Lek. 2002;59(10):826-31. Przegl Lek. 2002. PMID: 12632922 Review. Polish.
-
Tissue plasminogen activator as a modulator of neuronal survival and function.Biochem Soc Trans. 2002 Apr;30(2):222-5. Biochem Soc Trans. 2002. PMID: 12023855 Review.
Cited by
-
One-Compound-Multi-Target: Combination Prospect of Natural Compounds with Thrombolytic Therapy in Acute Ischemic Stroke.Curr Neuropharmacol. 2017;15(1):134-156. doi: 10.2174/1570159x14666160620102055. Curr Neuropharmacol. 2017. PMID: 27334020 Free PMC article. Review.
-
Tissue Plasminogen Activator Loaded PCL Nanofibrous Scaffold Promoted Nerve Regeneration After Sciatic Nerve Transection in Male Rats.Neurotox Res. 2021 Apr;39(2):413-428. doi: 10.1007/s12640-020-00276-z. Epub 2020 Aug 27. Neurotox Res. 2021. PMID: 32852719
-
Basement membrane and blood-brain barrier.Stroke Vasc Neurol. 2018 Dec 5;4(2):78-82. doi: 10.1136/svn-2018-000198. eCollection 2019 Jul. Stroke Vasc Neurol. 2018. PMID: 31338215 Free PMC article. Review.
-
Presymptomatic activation of the PDGF-CC pathway accelerates onset of ALS neurodegeneration.Acta Neuropathol. 2016 Mar;131(3):453-64. doi: 10.1007/s00401-015-1520-2. Epub 2015 Dec 19. Acta Neuropathol. 2016. PMID: 26687981 Free PMC article.
-
Role of mesenchymal stem cell-derived fibrinolytic factor in tissue regeneration and cancer progression.Cell Mol Life Sci. 2015 Dec;72(24):4759-70. doi: 10.1007/s00018-015-2035-7. Epub 2015 Sep 9. Cell Mol Life Sci. 2015. PMID: 26350342 Free PMC article. Review.
References
-
- Aase K, Abramsson A, Karlsson L, Betsholtz C, Eriksson U (2002) Expression analysis of PDGF-C in adult and developing mouse tissues. Mech Dev 110: 187–191 - PubMed
-
- Andrae J, Molander C, Smits A, Funa K, Nister M (2002) Platelet-derived growth factor-B and -C and active α-receptors in medulloblastoma cells. Biochem Biophys Res Commun 296: 604–611 - PubMed
-
- Bergsten E, Uutela M, Li X, Pietras K, Östman A, Heldin CH, Alitalo K, Eriksson U (2001) PDGF-D is a specific, protease-activated ligand for the PDGF β-receptor. Nat Cell Biol 3: 512–516 - PubMed
-
- Boucher P, Gotthardt M, Li WP, Anderson RG, Herz J (2003) LRP: role in vascular wall integrity and protection from atherosclerosis. Science 300: 329–332 - PubMed
-
- Bradford M (1976) A rapid and sensitive method for the quantification of microgram quantities of protein utilizing the principle of protein–dye binding. Anal Biochem 72: 248–254 - PubMed
Publication types
MeSH terms
Substances
LinkOut - more resources
Full Text Sources
Other Literature Sources
Molecular Biology Databases