In vivo investigation of the transcription, processing, endonucleolytic activity, and functional relevance of the spatial distribution of a plant miRNA
- PMID: 15371337
- PMCID: PMC517516
- DOI: 10.1101/gad.307804
In vivo investigation of the transcription, processing, endonucleolytic activity, and functional relevance of the spatial distribution of a plant miRNA
Erratum in
-
Corrigendum: In vivo investigation of the transcription, processing, endonucleolytic activity, and functional relevance of the spatial distribution of a plant miRNA.Genes Dev. 2015 Feb 15;29(4):465. Genes Dev. 2015. PMID: 25691472 Free PMC article. No abstract available.
-
Corrigendum: In vivo investigation of the transcription, processing, endonucleolytic activity, and functional relevance of the spatial distribution of a plant miRNA.Genes Dev. 2016 May 15;30(10):1251-2. doi: 10.1101/gad.283721.116. Genes Dev. 2016. PMID: 27222518 Free PMC article. No abstract available.
Abstract
We show, with miR171, that plant miRNA genes are modular independent transcription units in which the fold-back pre-miRNA is sufficient for miRNA processing, and that the upstream region contains highly specific promoter elements. Processing depends on flanking sequences within the miRNA stem-loop precursor rather than the miRNA sequence itself, and mutations affecting target pairing at the center and 5' but not 3' region of the miRNA compromise its function in vivo. Inactivation of the SDE1 RNA-dependent-RNA-polymerase was mandatory for accurate representation of miRNA activity by sensor constructs in Arabidopsis. Work in sde1 background revealed a near-perfect spatial overlap between the patterns of miR171 transcription and activity, supporting the idea that plant miRNAs enable cell differentiation.
Figures
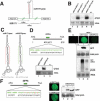
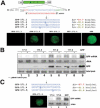
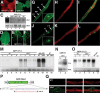
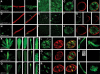
Similar articles
-
Structure determinants for accurate processing of miR172a in Arabidopsis thaliana.Curr Biol. 2010 Jan 12;20(1):42-8. doi: 10.1016/j.cub.2009.10.073. Curr Biol. 2010. PMID: 20015654
-
Identification of microRNA processing determinants by random mutagenesis of Arabidopsis MIR172a precursor.Curr Biol. 2010 Jan 12;20(1):49-54. doi: 10.1016/j.cub.2009.10.072. Epub 2009 Dec 10. Curr Biol. 2010. PMID: 20005105
-
RNA secondary structural determinants of miRNA precursor processing in Arabidopsis.Curr Biol. 2010 Jan 12;20(1):37-41. doi: 10.1016/j.cub.2009.10.076. Curr Biol. 2010. PMID: 20015653
-
New insights into pri-miRNA processing and accumulation in plants.Wiley Interdiscip Rev RNA. 2015 Sep-Oct;6(5):533-45. doi: 10.1002/wrna.1292. Epub 2015 Jun 29. Wiley Interdiscip Rev RNA. 2015. PMID: 26119101 Review.
-
SERRATE: a key factor in coordinated RNA processing in plants.Trends Plant Sci. 2023 Jul;28(7):841-853. doi: 10.1016/j.tplants.2023.03.009. Epub 2023 Apr 4. Trends Plant Sci. 2023. PMID: 37019716 Review.
Cited by
-
Drought-inducible expression of Hv-miR827 enhances drought tolerance in transgenic barley.Funct Integr Genomics. 2017 May;17(2-3):279-292. doi: 10.1007/s10142-016-0526-8. Epub 2016 Oct 11. Funct Integr Genomics. 2017. PMID: 27730426
-
Rice osa-miR171c Mediates Phase Change from Vegetative to Reproductive Development and Shoot Apical Meristem Maintenance by Repressing Four OsHAM Transcription Factors.PLoS One. 2015 May 29;10(5):e0125833. doi: 10.1371/journal.pone.0125833. eCollection 2015. PLoS One. 2015. PMID: 26023934 Free PMC article.
-
Cloning and characterization of microRNAs from wheat (Triticum aestivum L.).Genome Biol. 2007;8(6):R96. doi: 10.1186/gb-2007-8-6-r96. Genome Biol. 2007. PMID: 17543110 Free PMC article.
-
Cell signalling by microRNA165/6 directs gene dose-dependent root cell fate.Nature. 2010 May 20;465(7296):316-21. doi: 10.1038/nature08977. Epub 2010 Apr 21. Nature. 2010. PMID: 20410882 Free PMC article.
-
Shoot meristem function and leaf polarity: the role of class III HD-ZIP genes.PLoS Genet. 2006 Jun;2(6):e89. doi: 10.1371/journal.pgen.0020089. PLoS Genet. 2006. PMID: 16846251 Free PMC article. Review.
References
-
- Bartel D.P. 2004. MicroRNAs: Genomics, biogenesis, mechanism, and function. Cell 116: 281-297. - PubMed
-
- Bechtold N. and Pelletier, G. 1998. In planta Agrobacterium-mediated transformation of adult Arabidopsis thaliana plants by vacuum infiltration. Methods Mol. Biol. 82: 259-266. - PubMed
-
- Bernstein E., Kim, S.Y., Carmell, M.A., Murchison, E.P., Alcorn H., Li, M.Z., Mills, A.A., Elledge, S.J., Anderson, K.V., and Hannon, G.J. 2003. Dicer is essential for mouse development. Nat. Genet. 35: 215-217. - PubMed
Publication types
MeSH terms
Substances
LinkOut - more resources
Full Text Sources
Other Literature Sources
Molecular Biology Databases