FANCG is phosphorylated at serines 383 and 387 during mitosis
- PMID: 15367677
- PMCID: PMC516759
- DOI: 10.1128/MCB.24.19.8576-8585.2004
FANCG is phosphorylated at serines 383 and 387 during mitosis
Abstract
Fanconi anemia (FA) is an autosomal recessive disease marked by congenital defects, bone marrow failure, and high incidence of leukemia and solid tumors. Eight genes have been cloned, with the accompanying protein products participating in at least two complexes, which appear to be functionally dependent upon one another. Previous studies have described chromatin localization of the FA core complex, except at mitosis, which is associated with phosphorylation of the FANCG protein (F. Qiao, A. Moss, and G. M. Kupfer, J. Biol. Chem. 276:23391-23396, 2001). The phosphorylation of FANCG at serine 7 by using mass spectrometry was previously mapped. The purpose of this study was to map the phosphorylation sites of FANCG at mitosis and to assess their functional importance. Reasoning that a potential kinase might be cdc2, which was previously reported to bind to FANCC, we showed that cdc2 chiefly phosphorylated a 14-kDa fragment of the C-terminal half of FANCG. Mass spectrometry analysis demonstrated that this fragment contains amino acids 374 to 504. Kinase motif analysis demonstrated that three amino acids in this fragment were leading candidates for phosphorylation. By using PCR-directed in vitro mutagenesis we mutated S383, S387, and T487 to alanine. Mutation of S383 and S387 abolished the phosphorylation of FANCG at mitosis. These results were confirmed by use of phosphospecific antibodies directed against phosphoserine 383 and phosphoserine 387. Furthermore, the ability to correct FA-G mutant cells of human or hamster (where S383 and S387 are conserved) origin was also impaired by these mutations, demonstrating the functional importance of these amino acids. S387A mutant abolished FANCG fusion protein phosphorylation by cdc2. The FA pathway, of which FANCG is a part, is highly regulated by a series of phosphorylation steps that are important to its overall function.
Figures
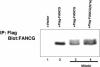
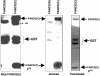
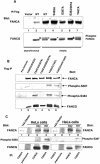
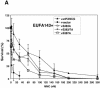
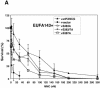
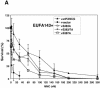
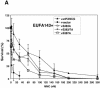
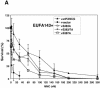
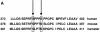
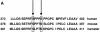
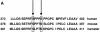
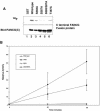
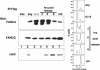
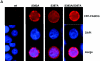
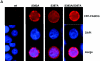
Similar articles
-
Phosphorylation of fanconi anemia (FA) complementation group G protein, FANCG, at serine 7 is important for function of the FA pathway.J Biol Chem. 2004 Oct 29;279(44):46035-45. doi: 10.1074/jbc.M408323200. Epub 2004 Aug 6. J Biol Chem. 2004. PMID: 15299017
-
FANCG promotes formation of a newly identified protein complex containing BRCA2, FANCD2 and XRCC3.Oncogene. 2008 Jun 12;27(26):3641-52. doi: 10.1038/sj.onc.1211034. Epub 2008 Jan 21. Oncogene. 2008. PMID: 18212739
-
The Fanconi anemia core complex forms four complexes of different sizes in different subcellular compartments.J Biol Chem. 2004 Jun 18;279(25):26201-9. doi: 10.1074/jbc.M400091200. Epub 2004 Apr 13. J Biol Chem. 2004. PMID: 15082718
-
Tetratricopeptide-motif-mediated interaction of FANCG with recombination proteins XRCC3 and BRCA2.DNA Repair (Amst). 2006 May 10;5(5):629-40. doi: 10.1016/j.dnarep.2006.02.007. Epub 2006 Apr 18. DNA Repair (Amst). 2006. PMID: 16621732
-
Resistance to mitomycin C requires direct interaction between the Fanconi anemia proteins FANCA and FANCG in the nucleus through an arginine-rich domain.J Biol Chem. 1999 Nov 26;274(48):34212-8. doi: 10.1074/jbc.274.48.34212. J Biol Chem. 1999. PMID: 10567393
Cited by
-
Fanconi anemia: a signal transduction and DNA repair pathway.Yale J Biol Med. 2013 Dec 13;86(4):491-7. Yale J Biol Med. 2013. PMID: 24348213 Free PMC article. Review.
-
Fanconi anemia signaling network regulates the spindle assembly checkpoint.J Clin Invest. 2013 Sep;123(9):3839-47. doi: 10.1172/JCI67364. Epub 2013 Aug 15. J Clin Invest. 2013. PMID: 23934222 Free PMC article.
-
Fanconi anemia.Hematol Oncol Clin North Am. 2009 Apr;23(2):193-214. doi: 10.1016/j.hoc.2009.01.008. Hematol Oncol Clin North Am. 2009. PMID: 19327579 Free PMC article. Review.
-
UBE2T, the Fanconi anemia core complex, and FANCD2 are recruited independently to chromatin: a basis for the regulation of FANCD2 monoubiquitination.Mol Cell Biol. 2007 Dec;27(24):8421-30. doi: 10.1128/MCB.00504-07. Epub 2007 Oct 15. Mol Cell Biol. 2007. PMID: 17938197 Free PMC article.
-
The Fanconi anemia pathway and ICL repair: implications for cancer therapy.Crit Rev Biochem Mol Biol. 2010 Oct;45(5):424-39. doi: 10.3109/10409238.2010.502166. Crit Rev Biochem Mol Biol. 2010. PMID: 20807115 Free PMC article. Review.
References
-
- Adachi, D., T. Oda, H. Yagasaki, K. Nakasato, T. Taniguchi, A. D. D'Andrea, S. Asano, and T. Yamashita. 2002. Heterogeneous activation of the Fanconi anemia pathway by patient-derived FANCA mutants. Hum. Mol. Genet. 11:3125-3134. - PubMed
-
- Alter, B. P., and N. S. Young. 1993. The bone marrow failure syndromes, p. 216-316. In D. G. Nathan and F. A. Oski (ed.), Hematology of infancy and childhood, vol. 1. W.B. Saunders, Philadelphia, Pa.
-
- Apostolou, S., S. A. Whitmore, J. Crawford, et al. 1996. Positional cloning of the Fanconi anaemia group A gene. Nat. Genet. 14:324-328. - PubMed
-
- Auerbach, A., M. Buchwald, and H. Joenje. 1997. Fanconi anemia, p. 317-332. In B. Vogelstein and K. Kinzler (ed.), Genetics of cancer. McGraw-Hill, New York, N.Y.
Publication types
MeSH terms
Substances
Grants and funding
LinkOut - more resources
Full Text Sources
Molecular Biology Databases
Research Materials
Miscellaneous