Calpain mediates calcium-induced activation of the erk1,2 MAPK pathway and cytoskeletal phosphorylation in neurons: relevance to Alzheimer's disease
- PMID: 15331404
- PMCID: PMC1618589
- DOI: 10.1016/S0002-9440(10)63342-1
Calpain mediates calcium-induced activation of the erk1,2 MAPK pathway and cytoskeletal phosphorylation in neurons: relevance to Alzheimer's disease
Abstract
Aberrant phosphorylation of the neuronal cytoskeleton is an early pathological event in Alzheimer's disease (AD), but the underlying mechanisms are unclear. Here, we demonstrate in the brains of AD patients that neurofilament hyperphosphorylation in neocortical pyramidal neurons is accompanied by activation of both Erk1,2 and calpain. Using immunochemistry, Western blot analysis, and kinase activity measurements, we show in primary hippocampal and cerebellar granule (CG) neurons that calcium influx activates calpain and Erk1,2 and increases neurofilament phosphorylation on carboxy terminal polypeptide sites known to be modulated by Erk1,2 and to be altered in AD. Blocking Erk1,2 activity either with antisense oligonucleotides to Erk1,2 mRNA sequences or by specifically inhibiting its upstream activating kinase MEK1,2 markedly reduced neurofilament phosphorylation. Calpeptin, a cell-permeable calpain inhibitor, blocked both Erk1,2 activation and neurofilament hyperphosphorylation at concentrations that inhibit calpain-mediated cleavage of brain spectrin. By contrast, inhibiting Erk1,2 with U-0126, a specific inhibitor of Mek1,2, had no appreciable effect on ionomycin-induced calpain activation. These findings demonstrate that, under conditions of calcium injury in neurons, calpains are upstream activators of Erk1,2 signaling and are likely to mediate in part the hyperphosphorylation of neurofilaments and tau seen at early stages of AD as well as the neuron survival-related functions of the MAP kinase pathway.
Figures
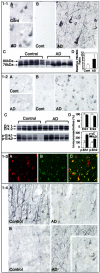
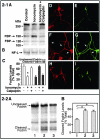
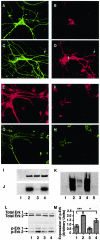
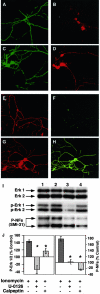
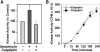
Similar articles
-
Comparison of Two Modern Survival Prediction Tools, SORG-MLA and METSSS, in Patients With Symptomatic Long-bone Metastases Who Underwent Local Treatment With Surgery Followed by Radiotherapy and With Radiotherapy Alone.Clin Orthop Relat Res. 2024 Dec 1;482(12):2193-2208. doi: 10.1097/CORR.0000000000003185. Epub 2024 Jul 23. Clin Orthop Relat Res. 2024. PMID: 39051924
-
Depressing time: Waiting, melancholia, and the psychoanalytic practice of care.In: Kirtsoglou E, Simpson B, editors. The Time of Anthropology: Studies of Contemporary Chronopolitics. Abingdon: Routledge; 2020. Chapter 5. In: Kirtsoglou E, Simpson B, editors. The Time of Anthropology: Studies of Contemporary Chronopolitics. Abingdon: Routledge; 2020. Chapter 5. PMID: 36137063 Free Books & Documents. Review.
-
Protein tau phosphorylation in the proline rich region and its implication in the progression of Alzheimer's disease.Exp Neurol. 2025 Jan;383:115049. doi: 10.1016/j.expneurol.2024.115049. Epub 2024 Nov 8. Exp Neurol. 2025. PMID: 39522802
-
Tobacco-specific nitrosamine 4-(methylnitrosamino)-1-(3-pyridyl)-1-butanone induces phosphorylation of mu- and m-calpain in association with increased secretion, cell migration, and invasion.J Biol Chem. 2004 Dec 17;279(51):53683-90. doi: 10.1074/jbc.M409889200. Epub 2004 Oct 7. J Biol Chem. 2004. PMID: 15471877
-
Pharmacological treatments in panic disorder in adults: a network meta-analysis.Cochrane Database Syst Rev. 2023 Nov 28;11(11):CD012729. doi: 10.1002/14651858.CD012729.pub3. Cochrane Database Syst Rev. 2023. PMID: 38014714 Free PMC article. Review.
Cited by
-
The contributions of myelin and axonal caliber to transverse relaxation time in shiverer and neurofilament-deficient mouse models.Neuroimage. 2010 Jul 1;51(3):1098-105. doi: 10.1016/j.neuroimage.2010.03.013. Epub 2010 Mar 11. Neuroimage. 2010. PMID: 20226865 Free PMC article.
-
Viscoelastic Response of Neurofilaments: An Atomistic Simulation Approach.Biomolecules. 2021 Apr 7;11(4):540. doi: 10.3390/biom11040540. Biomolecules. 2021. PMID: 33917073 Free PMC article.
-
Zearalenone Induces Endothelial Cell Apoptosis through Activation of a Cytosolic Ca2+/ERK1/2/p53/Caspase 3 Signaling Pathway.Toxins (Basel). 2021 Mar 4;13(3):187. doi: 10.3390/toxins13030187. Toxins (Basel). 2021. PMID: 33806711 Free PMC article.
-
Early Life Stress and Epigenetics in Late-onset Alzheimer's Dementia: A Systematic Review.Curr Genomics. 2018 Nov;19(7):522-602. doi: 10.2174/1389202919666171229145156. Curr Genomics. 2018. PMID: 30386171 Free PMC article. Review.
-
Specific calpain inhibition by calpastatin prevents tauopathy and neurodegeneration and restores normal lifespan in tau P301L mice.J Neurosci. 2014 Jul 9;34(28):9222-34. doi: 10.1523/JNEUROSCI.1132-14.2014. J Neurosci. 2014. PMID: 25009256 Free PMC article.
References
-
- Julien J. Neurofilament functions in health and disease. Curr Opin Neurobiol. 1999;9:554–560. - PubMed
-
- Lavedan C, Buchholtz S, Nussbaum R, Albin R, Polymeropoulos M. A mutation in the human neurofilament M gene in Parkinson’s disease that suggests a role for the cytoskeleton in neuronal degeneration. Neurosci Lett. 2002;322:57–61. - PubMed
-
- Al-Chalabi A, Miller C. Neurofilaments and neurological disease. Bioessays. 2003;25:346–355. - PubMed
Publication types
MeSH terms
Substances
Grants and funding
LinkOut - more resources
Full Text Sources
Other Literature Sources
Medical
Miscellaneous