Activation of a vinculin-binding site in the talin rod involves rearrangement of a five-helix bundle
- PMID: 15272303
- PMCID: PMC514914
- DOI: 10.1038/sj.emboj.7600285
Activation of a vinculin-binding site in the talin rod involves rearrangement of a five-helix bundle
Abstract
The interaction between the cytoskeletal proteins talin and vinculin plays a key role in integrin-mediated cell adhesion and migration. We have determined the crystal structures of two domains from the talin rod spanning residues 482-789. Talin 482-655, which contains a vinculin-binding site (VBS), folds into a five-helix bundle whereas talin 656-789 is a four-helix bundle. We show that the VBS is composed of a hydrophobic surface spanning five turns of helix 4. All the key side chains from the VBS are buried and contribute to the hydrophobic core of the talin 482-655 fold. We demonstrate that the talin 482-655 five-helix bundle represents an inactive conformation, and mutations that disrupt the hydrophobic core or deletion of helix 5 are required to induce an active conformation in which the VBS is exposed. We also report the crystal structure of the N-terminal vinculin head domain in complex with an activated form of talin. Activation of the VBS in talin and the recruitment of vinculin may support the maturation of small integrin/talin complexes into more stable adhesions.
Figures
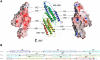
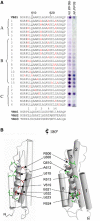
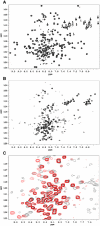
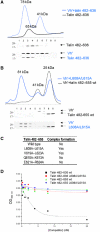
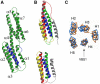
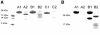
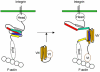
Similar articles
-
A vinculin binding domain from the talin rod unfolds to form a complex with the vinculin head.Structure. 2005 Jan;13(1):65-74. doi: 10.1016/j.str.2004.11.006. Structure. 2005. PMID: 15642262
-
Intermolecular versus intramolecular interactions of the vinculin binding site 33 of talin.Protein Sci. 2011 Aug;20(8):1471-6. doi: 10.1002/pro.671. Protein Sci. 2011. PMID: 21648001 Free PMC article.
-
Mapping and consensus sequence identification for multiple vinculin binding sites within the talin rod.J Biol Chem. 2005 Nov 4;280(44):37217-24. doi: 10.1074/jbc.M508060200. Epub 2005 Aug 30. J Biol Chem. 2005. PMID: 16135522
-
Integrin connections to the cytoskeleton through talin and vinculin.Biochem Soc Trans. 2008 Apr;36(Pt 2):235-9. doi: 10.1042/BST0360235. Biochem Soc Trans. 2008. PMID: 18363566 Review.
-
Cytoskeletal proteins talin and vinculin in integrin-mediated adhesion.Biochem Soc Trans. 2004 Nov;32(Pt 5):831-6. doi: 10.1042/BST0320831. Biochem Soc Trans. 2004. PMID: 15494027 Review.
Cited by
-
Multiscale force sensing in development.Nat Cell Biol. 2017 May 31;19(6):581-588. doi: 10.1038/ncb3524. Nat Cell Biol. 2017. PMID: 28561050 Review.
-
Cellular stiffness sensing through talin 1 in tissue mechanical homeostasis.Sci Adv. 2024 Aug 23;10(34):eadi6286. doi: 10.1126/sciadv.adi6286. Epub 2024 Aug 21. Sci Adv. 2024. PMID: 39167642 Free PMC article.
-
Phosphoinositides regulate force-independent interactions between talin, vinculin, and actin.Elife. 2020 Jul 13;9:e56110. doi: 10.7554/eLife.56110. Elife. 2020. PMID: 32657269 Free PMC article.
-
RIAM and vinculin binding to talin are mutually exclusive and regulate adhesion assembly and turnover.J Biol Chem. 2013 Mar 22;288(12):8238-8249. doi: 10.1074/jbc.M112.438119. Epub 2013 Feb 6. J Biol Chem. 2013. PMID: 23389036 Free PMC article.
-
The Architecture of Talin1 Reveals an Autoinhibition Mechanism.Cell. 2019 Sep 19;179(1):120-131.e13. doi: 10.1016/j.cell.2019.08.034. Cell. 2019. PMID: 31539492 Free PMC article.
References
-
- Albiges-Rizo C, Frachet P, Block MR (1995) Down regulation of talin alters cell adhesion and the processing of the α5β1 integrin. J Cell Sci 108: 3317–3329 - PubMed
-
- Bakolitsa C, dePereda JM, Bagshaw CR, Critchley DR, Liddington RC (1999) Crystal structure of the vinculin tail suggests a pathway for activation. Cell 99: 603–613 - PubMed
-
- Barsukov IL, Prescot A, Bate N, Patel B, Floyd DN, Bhanji N, Bagshaw CR, Letinic K, Di Paolo G, De Camilli P, Roberts GC, Critchley DR (2003) Phosphatidylinositol phosphate kinase type 1gamma and beta1-integrin cytoplasmic domain bind to the same region in the talin FERM domain. J Biol Chem 278: 31202–31209 - PubMed
Publication types
MeSH terms
Substances
Associated data
- Actions
- Actions
- Actions
LinkOut - more resources
Full Text Sources
Other Literature Sources
Molecular Biology Databases