RNA editing of a miRNA precursor
- PMID: 15272117
- PMCID: PMC1370607
- DOI: 10.1261/rna.7350304
RNA editing of a miRNA precursor
Abstract
Micro RNAs comprise a large family of small, functional RNAs with important roles in the regulation of protein coding genes in animals and plants. Here we show that human and mouse miRNA22 precursor molecules are subject to posttranscriptional modification by A-to-I RNA editing in vivo. The observed editing events are predicted to have significant implications for the biogenesis and function of miRNA22 and might point toward a more general role for RNA editing in the regulation of miRNA gene expression.
Figures
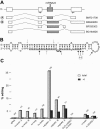
Similar articles
-
MiRNA editing.Methods Mol Biol. 2010;667:267-79. doi: 10.1007/978-1-60761-811-9_18. Methods Mol Biol. 2010. PMID: 20827540
-
Using synthetic precursor and inhibitor miRNAs to understand miRNA function.Methods Mol Biol. 2008;419:289-301. doi: 10.1007/978-1-59745-033-1_20. Methods Mol Biol. 2008. PMID: 18369991
-
ADAR2-mediated editing of RNA substrates in the nucleolus is inhibited by C/D small nucleolar RNAs.J Cell Biol. 2005 Jun 6;169(5):745-53. doi: 10.1083/jcb.200411129. J Cell Biol. 2005. PMID: 15939761 Free PMC article.
-
[Regulation of the miRNA function by RNA editing].Tanpakushitsu Kakusan Koso. 2009 Jul;54(9):1133-40. Tanpakushitsu Kakusan Koso. 2009. PMID: 19588876 Review. Japanese. No abstract available.
-
Gene regulation through RNA editing.Discov Med. 2010 Nov;10(54):379-86. Discov Med. 2010. PMID: 21122469 Review.
Cited by
-
The use of high-throughput sequencing methods for plant microRNA research.RNA Biol. 2015;12(7):709-19. doi: 10.1080/15476286.2015.1053686. RNA Biol. 2015. PMID: 26016494 Free PMC article. Review.
-
RNA editing in the human ENCODE RNA-seq data.Genome Res. 2012 Sep;22(9):1626-33. doi: 10.1101/gr.134957.111. Genome Res. 2012. PMID: 22955975 Free PMC article.
-
Regulation of small RNA stability: methylation and beyond.Cell Res. 2012 Apr;22(4):624-36. doi: 10.1038/cr.2012.36. Epub 2012 Mar 13. Cell Res. 2012. PMID: 22410795 Free PMC article. Review.
-
MicroRNAs and DNA damage response: implications for cancer therapy.Cell Cycle. 2013 Jan 1;12(1):32-42. doi: 10.4161/cc.23051. Epub 2012 Dec 19. Cell Cycle. 2013. PMID: 23255103 Free PMC article. Review.
-
A mammalian microRNA expression atlas based on small RNA library sequencing.Cell. 2007 Jun 29;129(7):1401-14. doi: 10.1016/j.cell.2007.04.040. Cell. 2007. PMID: 17604727 Free PMC article.
References
-
- Bartel, D.P. 2004. MicroRNAs: Genomics, biogenesis, mechanism, and function. Cell 116: 281–297. - PubMed
-
- Bernstein, E., Caudy, A.A., Hammond, S.M., and Hannon, G.J. 2001. Role for a bidentate ribonuclease in the initiation step of RNA interference. Nature 409: 363–366. - PubMed
-
- Boguski, M.S., Lowe, T.M., and Tolstoshev, C.M. 1993. dbEST—Database for “expressed sequence tags.” Nat. Genet. 4: 332–333. - PubMed
-
- Burns, C.M., Chu, H., Rueter, S.M., Hutchinson, L.K., Canton, H., Sanders-Bush, E., and Emeson, R.B. 1997. Regulation of serotonin-2C receptor G-protein coupling by RNA editing [see comments]. Nature 387: 303–308. - PubMed
Publication types
MeSH terms
Substances
LinkOut - more resources
Full Text Sources
Other Literature Sources