Non-selective cationic channels of smooth muscle and the mammalian homologues of Drosophila TRP
- PMID: 15272031
- PMCID: PMC1665181
- DOI: 10.1113/jphysiol.2004.068734
Non-selective cationic channels of smooth muscle and the mammalian homologues of Drosophila TRP
Erratum in
- J Physiol. 2004 Nov 1;560(Pt 3):949
Abstract
Throughout the body there are smooth muscle cells controlling a myriad of tubes and reservoirs. The cells show enormous diversity and complexity compounded by a plasticity that is critical in physiology and disease. Over the past quarter of a century we have seen that smooth muscle cells contain--as part of a gamut of ion-handling mechanisms--a family of cationic channels with significant permeability to calcium, potassium and sodium. Several of these channels are sensors of calcium store depletion, G-protein-coupled receptor activation, membrane stretch, intracellular Ca2+, pH, phospholipid signals and other factors. Progress in understanding the channels has, however, been hampered by a paucity of specific pharmacological agents and difficulty in identifying the underlying genes. In this review we summarize current knowledge of these smooth muscle cationic channels and evaluate the hypothesis that the underlying genes are homologues of Drosophila TRP (transient receptor potential). Direct evidence exists for roles of TRPC1, TRPC4/5, TRPC6, TRPV2, TRPP1 and TRPP2, and more are likely to be added soon. Some of these TRP proteins respond to a multiplicity of activation signals--promiscuity of gating that could enable a variety of context-dependent functions. We would seem to be witnessing the first phase of the molecular delineation of these cationic channels, something that should prove a leap forward for strategies aimed at developing new selective pharmacological agents and understanding the activation mechanisms and functions of these channels in physiological systems.
Figures
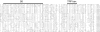
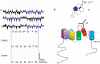
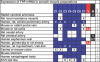
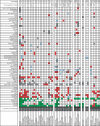
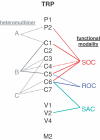
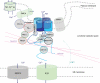
Similar articles
-
Emerging functions of 10 types of TRP cationic channel in vascular smooth muscle.Clin Exp Pharmacol Physiol. 2005 Aug;32(8):597-603. doi: 10.1111/j.1440-1681.2005.04251.x. Clin Exp Pharmacol Physiol. 2005. PMID: 16120184 Free PMC article. Review.
-
Cation channels of the transient receptor potential superfamily: their role in physiological and pathophysiological processes of smooth muscle cells.Pharmacol Ther. 2006 Dec;112(3):744-60. doi: 10.1016/j.pharmthera.2006.05.013. Epub 2006 Jul 13. Pharmacol Ther. 2006. PMID: 16842858 Review.
-
Transient receptor potential ion channels and animal sensation: lessons from Drosophila functional research.J Biochem Mol Biol. 2004 Jan 31;37(1):114-21. doi: 10.5483/bmbrep.2004.37.1.114. J Biochem Mol Biol. 2004. PMID: 14761309 Review.
-
Molecular and functional characterization of a novel mouse transient receptor potential protein homologue TRP7. Ca(2+)-permeable cation channel that is constitutively activated and enhanced by stimulation of G protein-coupled receptor.J Biol Chem. 1999 Sep 24;274(39):27359-70. doi: 10.1074/jbc.274.39.27359. J Biol Chem. 1999. PMID: 10488066
-
TRPC1, a human homolog of a Drosophila store-operated channel.Proc Natl Acad Sci U S A. 1995 Oct 10;92(21):9652-6. doi: 10.1073/pnas.92.21.9652. Proc Natl Acad Sci U S A. 1995. PMID: 7568191 Free PMC article.
Cited by
-
Angiotensin II activates two cation conductances with distinct TRPC1 and TRPC6 channel properties in rabbit mesenteric artery myocytes.J Physiol. 2006 Dec 1;577(Pt 2):479-95. doi: 10.1113/jphysiol.2006.119305. Epub 2006 Sep 14. J Physiol. 2006. PMID: 16973707 Free PMC article.
-
Effects of the muscarinic agonist, 5-methylfurmethiodide, on contraction and electrophysiology of Ascaris suum muscle.Int J Parasitol. 2008 Jul;38(8-9):945-57. doi: 10.1016/j.ijpara.2007.11.011. Epub 2007 Dec 8. Int J Parasitol. 2008. PMID: 18206155 Free PMC article.
-
Interactions, functions, and independence of plasma membrane STIM1 and TRPC1 in vascular smooth muscle cells.Circ Res. 2008 Oct 10;103(8):e97-104. doi: 10.1161/CIRCRESAHA.108.182931. Epub 2008 Sep 18. Circ Res. 2008. PMID: 18802022 Free PMC article.
-
Activation of c-SRC underlies the differential effects of ouabain and digoxin on Ca(2+) signaling in arterial smooth muscle cells.Am J Physiol Cell Physiol. 2013 Feb 15;304(4):C324-33. doi: 10.1152/ajpcell.00337.2012. Epub 2012 Nov 28. Am J Physiol Cell Physiol. 2013. PMID: 23195071 Free PMC article.
-
Region-specific changes in transient receptor potential vanilloid channel expression in the vasopressin magnocellular system in hepatic cirrhosis-induced hyponatraemia.J Neuroendocrinol. 2012 Apr;24(4):642-52. doi: 10.1111/j.1365-2826.2011.02273.x. J Neuroendocrinol. 2012. PMID: 22188460 Free PMC article.
References
-
- Ahmmed GU, Mehta D, Vogel S, Holinstat M, Paria BC, Tiruppathi C, Malik AB. Protein kinase C-α phosphorylates the TRPC1 channel and regulates store-operated Ca2+ entry in endothelial cells. J Biol Chem. 2004;279:20941–20949. - PubMed
Publication types
MeSH terms
Substances
Grants and funding
LinkOut - more resources
Full Text Sources
Molecular Biology Databases
Miscellaneous