Mitosis-specific anchoring of gamma tubulin complexes by pericentrin controls spindle organization and mitotic entry
- PMID: 15146056
- PMCID: PMC491825
- DOI: 10.1091/mbc.e03-11-0796
Mitosis-specific anchoring of gamma tubulin complexes by pericentrin controls spindle organization and mitotic entry
Abstract
Microtubule nucleation is the best known function of centrosomes. Centrosomal microtubule nucleation is mediated primarily by gamma tubulin ring complexes (gamma TuRCs). However, little is known about the molecules that anchor these complexes to centrosomes. In this study, we show that the centrosomal coiled-coil protein pericentrin anchors gamma TuRCs at spindle poles through an interaction with gamma tubulin complex proteins 2 and 3 (GCP2/3). Pericentrin silencing by small interfering RNAs in somatic cells disrupted gamma tubulin localization and spindle organization in mitosis but had no effect on gamma tubulin localization or microtubule organization in interphase cells. Similarly, overexpression of the GCP2/3 binding domain of pericentrin disrupted the endogenous pericentrin-gamma TuRC interaction and perturbed astral microtubules and spindle bipolarity. When added to Xenopus mitotic extracts, this domain uncoupled gamma TuRCs from centrosomes, inhibited microtubule aster assembly, and induced rapid disassembly of preassembled asters. All phenotypes were significantly reduced in a pericentrin mutant with diminished GCP2/3 binding and were specific for mitotic centrosomal asters as we observed little effect on interphase asters or on asters assembled by the Ran-mediated centrosome-independent pathway. Additionally, pericentrin silencing or overexpression induced G2/antephase arrest followed by apoptosis in many but not all cell types. We conclude that pericentrin anchoring of gamma tubulin complexes at centrosomes in mitotic cells is required for proper spindle organization and that loss of this anchoring mechanism elicits a checkpoint response that prevents mitotic entry and triggers apoptotic cell death.
Figures
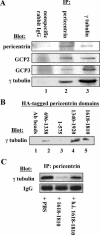
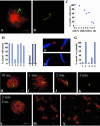
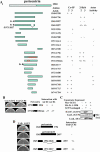
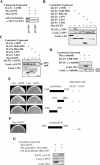
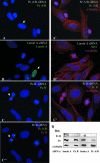
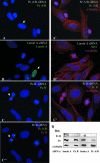
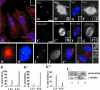
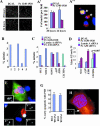
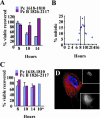
Similar articles
-
A fraction of Crm1 locates at centrosomes by its CRIME domain and regulates the centrosomal localization of pericentrin.Biochem Biophys Res Commun. 2009 Jul 3;384(3):383-8. doi: 10.1016/j.bbrc.2009.04.154. Epub 2009 May 5. Biochem Biophys Res Commun. 2009. PMID: 19422798
-
TACC3-ch-TOG interaction regulates spindle microtubule assembly by controlling centrosomal recruitment of γ-TuRC.Biosci Rep. 2023 Mar 29;43(3):BSR20221882. doi: 10.1042/BSR20221882. Biosci Rep. 2023. PMID: 36790370 Free PMC article.
-
Aurora A site specific TACC3 phosphorylation regulates astral microtubule assembly by stabilizing γ-tubulin ring complex.BMC Mol Cell Biol. 2019 Dec 10;20(1):58. doi: 10.1186/s12860-019-0242-z. BMC Mol Cell Biol. 2019. PMID: 31823729 Free PMC article.
-
Regulation of microtubule nucleation mediated by γ-tubulin complexes.Protoplasma. 2017 May;254(3):1187-1199. doi: 10.1007/s00709-016-1070-z. Epub 2017 Jan 10. Protoplasma. 2017. PMID: 28074286 Review.
-
Centrosomal and Non-centrosomal Functions Emerged through Eliminating Centrosomes.Cell Struct Funct. 2020 May 23;45(1):57-64. doi: 10.1247/csf.20007. Epub 2020 Apr 9. Cell Struct Funct. 2020. PMID: 32269206 Free PMC article. Review.
Cited by
-
Subcellular Localization and Mitotic Interactome Analyses Identify SIRT4 as a Centrosomally Localized and Microtubule Associated Protein.Cells. 2020 Aug 24;9(9):1950. doi: 10.3390/cells9091950. Cells. 2020. PMID: 32846968 Free PMC article.
-
Dysregulation of the endoplasmic reticulum blocks recruitment of centrosome-associated proteins resulting in mitotic failure.Development. 2023 Nov 15;150(22):dev201917. doi: 10.1242/dev.201917. Epub 2023 Nov 17. Development. 2023. PMID: 37971218 Free PMC article.
-
Silencing of Taxol-Sensitizer Genes in Cancer Cells: Lack of Sensitization Effects.Cancers (Basel). 2015 Jun 16;7(2):1052-71. doi: 10.3390/cancers7020824. Cancers (Basel). 2015. PMID: 26086592 Free PMC article.
-
RNAs induced by Epstein-Barr virus nuclear antigen 2 in lymphoblastoid cell lines.Proc Natl Acad Sci U S A. 2006 Feb 7;103(6):1900-5. doi: 10.1073/pnas.0510612103. Epub 2006 Jan 30. Proc Natl Acad Sci U S A. 2006. PMID: 16446431 Free PMC article.
-
A novel role for the centrosomal protein, pericentrin, in regulation of insulin secretory vesicle docking in mouse pancreatic beta-cells.PLoS One. 2010 Jul 27;5(7):e11812. doi: 10.1371/journal.pone.0011812. PLoS One. 2010. PMID: 20676397 Free PMC article.
References
-
- Barbosa, V., Gatt, M., Rebollo, E., Gonzalez, C., and Glover, D.M. (2003). Drosophila dd4 mutants reveal that gammaTuRC is required to maintain juxtaposed half spindles in spermatocytes. J. Cell Sci. 116, 929-941. - PubMed
-
- Blagden, S.P., and Glover, D.M. (2003). Polar expeditions–provisioning the centrosome for mitosis. Nat. Cell Biol. 5, 505-511. - PubMed
-
- Casenghi, M., Meraldi, P., Weinhart, U., Duncan, P.I., Korner, R., and Nigg, E.A. (2003). Polo-like kinase 1 regulates Nlp, a centrosome protein involved in microtubule nucleation. Dev. Cell. 5, 113-125. - PubMed
Publication types
MeSH terms
Substances
Grants and funding
LinkOut - more resources
Full Text Sources
Other Literature Sources
Miscellaneous