Intracellular topology and epitope shielding of poliovirus 3A protein
- PMID: 15140995
- PMCID: PMC415829
- DOI: 10.1128/JVI.78.11.5973-5982.2004
Intracellular topology and epitope shielding of poliovirus 3A protein
Abstract
The poliovirus RNA replication complex comprises multiple viral and possibly cellular proteins assembled on the cytoplasmic surface of rearranged intracellular membranes. Viral proteins 3A and 3AB perform several functions during the poliovirus replicative cycle, including significant roles in rearranging membranes, anchoring the viral polymerase to these membranes, inhibiting host protein secretion, and possibly providing the 3B protein primer for RNA synthesis. During poliovirus infection, the immunofluorescence signal of an amino-terminal epitope of 3A-containing proteins is markedly shielded compared to 3A protein expressed in the absence of other poliovirus proteins. This is not due to luminal orientation of all or a subset of the 3A-containing polypeptides, as shown by immunofluorescence following differential permeabilization and proteolysis experiments. Shielding of the 3A epitope is more pronounced in cells infected with wild-type poliovirus than in cells with temperature-sensitive mutant virus that contains a mutation in the 3D polymerase coding region adjacent to the 3AB binding site. Therefore, it is likely that direct binding of the poliovirus RNA-dependent RNA polymerase occludes the amino terminus of 3A-containing polypeptides in the RNA replication complex.
Figures
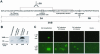
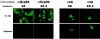
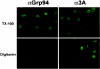
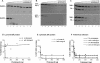
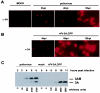
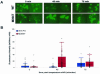
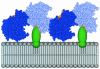
Similar articles
-
Inhibition of cellular protein secretion by picornaviral 3A proteins.Virology. 2005 Jun 20;337(1):18-29. doi: 10.1016/j.virol.2005.03.036. Virology. 2005. PMID: 15914217
-
Poliovirus RNA polymerase mutation 3D-M394T results in a temperature-sensitive defect in RNA synthesis.Virology. 1996 Mar 15;217(2):459-69. doi: 10.1006/viro.1996.0140. Virology. 1996. PMID: 8610437
-
Studies on the attenuation phenotype of polio vaccines: poliovirus RNA polymerase derived from Sabin type 1 sequence is temperature sensitive in the uridylylation of VPg.Virology. 2000 Jun 20;272(1):72-84. doi: 10.1006/viro.2000.0354. Virology. 2000. PMID: 10873750
-
Mapping of protein domains of hepatitis A virus 3AB essential for interaction with 3CD and viral RNA.Virology. 1999 Nov 25;264(2):410-21. doi: 10.1006/viro.1999.0017. Virology. 1999. PMID: 10562502
-
Paradoxes of the replication of picornaviral genomes.Virus Res. 1999 Aug;62(2):129-47. doi: 10.1016/s0168-1702(99)00037-4. Virus Res. 1999. PMID: 10507323 Review.
Cited by
-
Membrane topology and cellular dynamics of foot-and-mouth disease virus 3A protein.PLoS One. 2014 Oct 2;9(9):e106685. doi: 10.1371/journal.pone.0106685. eCollection 2014. PLoS One. 2014. PMID: 25275544 Free PMC article.
-
Mycovirus cryphonectria hypovirus 1 elements cofractionate with trans-Golgi network membranes of the fungal host Cryphonectria parasitica.J Virol. 2006 Jul;80(13):6588-96. doi: 10.1128/JVI.02519-05. J Virol. 2006. PMID: 16775345 Free PMC article.
-
Mutations that hamper dimerization of foot-and-mouth disease virus 3A protein are detrimental for infectivity.J Virol. 2012 Oct;86(20):11013-23. doi: 10.1128/JVI.00580-12. Epub 2012 Jul 11. J Virol. 2012. PMID: 22787230 Free PMC article.
-
Infectious Bursal Disease Virus Hijacks Endosomal Membranes as the Scaffolding Structure for Viral Replication.J Virol. 2018 May 14;92(11):e01964-17. doi: 10.1128/JVI.01964-17. Print 2018 Jun 1. J Virol. 2018. PMID: 29540593 Free PMC article.
-
ATP1B3: a virus-induced host factor against EV71 replication by up-regulating the production of type-I interferons.Virology. 2016 Sep;496:28-34. doi: 10.1016/j.virol.2016.05.013. Epub 2016 May 27. Virology. 2016. PMID: 27240146 Free PMC article.
References
-
- Aldabe, R., A. Barco, and L. Carrasco. 1996. Membrane permeabilization by poliovirus proteins 2B and 2BC. J. Biol. Chem. 271:23134-23137. - PubMed
-
- Barton, D. J., B. J. Morasco, L. Eisner-Smerage, P. S. Collis, S. E. Diamond, M. J. Hewlett, M. A. Merchant, B. J. O'Donnell, and J. B. Flanegan. 1996. Poliovirus RNA polymerase mutation 3D-M394T results in a temperature-sensitive defect in RNA synthesis. Virology 217:459-469. - PubMed
-
- Beckers, C. J., D. S. Keller, and W. E. Balch. 1989. Preparation of semiintact Chinese hamster ovary cells for reconstitution of endoplasmic reticulum-to-Golgi transport in a cell-free system. Methods Cell Biol. 31:91-102. - PubMed
Publication types
MeSH terms
Substances
LinkOut - more resources
Full Text Sources