Dopamine and glutamate control area-restricted search behavior in Caenorhabditis elegans
- PMID: 14762140
- PMCID: PMC6793574
- DOI: 10.1523/JNEUROSCI.1569-03.2004
Dopamine and glutamate control area-restricted search behavior in Caenorhabditis elegans
Abstract
Area-restricted search (ARS) is a foraging strategy used by many animals to locate resources. The behavior is characterized by a time-dependent reduction in turning frequency after the last resource encounter. This maximizes the time spent in areas in which resources are abundant and extends the search to a larger area when resources become scarce. We demonstrate that dopaminergic and glutamatergic signaling contribute to the neural circuit controlling ARS in the nematode Caenorhabditis elegans. Ablation of dopaminergic neurons eliminated ARS behavior, as did application of the dopamine receptor antagonist raclopride. Furthermore, ARS was affected by mutations in the glutamate receptor subunits GLR-1 and GLR-2 and the EAT-4 glutamate vesicular transporter. Interestingly, preincubation on dopamine restored the behavior in worms with defective dopaminergic signaling, but not in glr-1, glr-2, or eat-4 mutants. This suggests that dopaminergic and glutamatergic signaling function in the same pathway to regulate turn frequency. Both GLR-1 and GLR-2 are expressed in the locomotory control circuit that modulates the direction of locomotion in response to sensory stimuli and the duration of forward movement during foraging. We propose a mechanism for ARS in C. elegans in which dopamine, released in response to food, modulates glutamatergic signaling in the locomotory control circuit, thus resulting in an increased turn frequency.
Figures
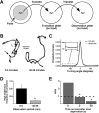
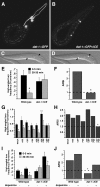
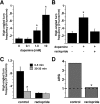
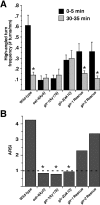
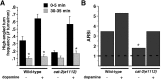
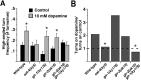
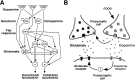
Similar articles
-
Dopamine modulation of avoidance behavior in Caenorhabditis elegans requires the NMDA receptor NMR-1.PLoS One. 2014 Aug 4;9(8):e102958. doi: 10.1371/journal.pone.0102958. eCollection 2014. PLoS One. 2014. PMID: 25089710 Free PMC article.
-
Multiple tracking and machine learning reveal dopamine modulation for area-restricted foraging behaviors via velocity change in Caenorhabditis elegans.Neurosci Lett. 2019 Jul 27;706:68-74. doi: 10.1016/j.neulet.2019.05.011. Epub 2019 May 10. Neurosci Lett. 2019. PMID: 31082452
-
The Snail transcription factor CES-1 regulates glutamatergic behavior in C. elegans.PLoS One. 2021 Feb 2;16(2):e0245587. doi: 10.1371/journal.pone.0245587. eCollection 2021. PLoS One. 2021. PMID: 33529210 Free PMC article.
-
Touch sensation in Caenorhabditis elegans.Bioessays. 1996 Mar;18(3):199-206. doi: 10.1002/bies.950180307. Bioessays. 1996. PMID: 8867734 Review.
-
Ionotropic glutamate receptors: genetics, behavior and electrophysiology.WormBook. 2006 Jan 19:1-16. doi: 10.1895/wormbook.1.61.1. WormBook. 2006. PMID: 18050468 Free PMC article. Review.
Cited by
-
Regulation of two motor patterns enables the gradual adjustment of locomotion strategy in Caenorhabditis elegans.Elife. 2016 May 25;5:e14116. doi: 10.7554/eLife.14116. Elife. 2016. PMID: 27222228 Free PMC article.
-
Haloperidol Interactions with the dop-3 Receptor in Caenorhabditis elegans.Mol Neurobiol. 2021 Jan;58(1):304-316. doi: 10.1007/s12035-020-02124-9. Epub 2020 Sep 15. Mol Neurobiol. 2021. PMID: 32935232
-
The Caenorhabditis elegans D2-like dopamine receptor DOP-2 physically interacts with GPA-14, a Gαi subunit.J Mol Signal. 2012 Jan 26;7(1):3. doi: 10.1186/1750-2187-7-3. J Mol Signal. 2012. PMID: 22280843 Free PMC article.
-
From modes to movement in the behavior of Caenorhabditis elegans.PLoS One. 2010 Nov 16;5(11):e13914. doi: 10.1371/journal.pone.0013914. PLoS One. 2010. PMID: 21103370 Free PMC article.
-
Food sensitizes C. elegans avoidance behaviours through acute dopamine signalling.EMBO J. 2011 Mar 16;30(6):1110-22. doi: 10.1038/emboj.2011.22. Epub 2011 Feb 8. EMBO J. 2011. PMID: 21304491 Free PMC article.
References
-
- Andow DA, Kareiva PM, Levin SA, Okubo A (1990) Spread of invading organisms. Landscape Ecol 4: 177–188.
-
- Avery L, Horvitz HR (1990) Effects of starvation and neuroactive drugs on feeding in Caenorhabditis elegans J Exp Zool 253: 263–270. - PubMed
-
- Bargmann CI, Horvitz HR (1991) Chemosensory neurons with overlapping functions direct chemotaxis to multiple chemicals in C. elegans Neuron 7: 729–742. - PubMed
-
- Bargmann CI, Hartwieg E, Horvitz HR (1993) Odorant-selective genes and neurons mediate olfaction in C. elegans Cell 74: 515–527. - PubMed
-
- Barnes S, Syed NI, Bulloch AG, Lukowiak K (1994) Modulation of ionic currents by dopamine in an interneurone of the respiratory central pattern generator of Lymnaea stagnalis J Exp Biol 189: 37–54. - PubMed
Publication types
MeSH terms
Substances
Grants and funding
LinkOut - more resources
Full Text Sources
Molecular Biology Databases