The zinc transporter ZnT3 interacts with AP-3 and it is preferentially targeted to a distinct synaptic vesicle subpopulation
- PMID: 14657250
- PMCID: PMC329249
- DOI: 10.1091/mbc.e03-06-0401
The zinc transporter ZnT3 interacts with AP-3 and it is preferentially targeted to a distinct synaptic vesicle subpopulation
Abstract
Synaptic vesicles (SV) are generated by two different mechanisms, one AP-2 dependent and one AP-3 dependent. It has been uncertain, however, whether these mechanisms generate SV that differ in molecular composition. We explored this hypothesis by analyzing the targeting of ZnT3 and synaptophysin both to PC12 synaptic-like microvesicles (SLMV) as well as SV isolated from wild-type and AP-3-deficient mocha brains. ZnT3 cytosolic tail interacted selectively with AP-3 in cell-free assays. Accordingly, pharmacological disruption of either AP-2- or AP-3-dependent SLMV biogenesis preferentially reduced synaptophysin or ZnT3 targeting, respectively; suggesting that these antigens were concentrated in different vesicles. As predicted, immuno-isolated SLMV revealed that ZnT3 and synaptophysin were enriched in different vesicle populations. Likewise, morphological and biochemical analyses in hippocampal neurons indicated that these two antigens were also present in distinct but overlapping domains. ZnT3 SV content was reduced in AP-3-deficient neurons, but synaptophysin was not altered in the AP-3 null background. Our evidence indicates that neuroendocrine cells assemble molecularly heterogeneous SV and suggests that this diversity could contribute to the functional variety of synapses.
Figures
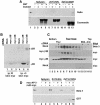
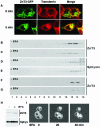
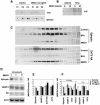
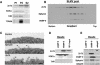
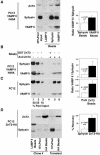
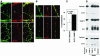
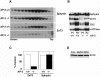
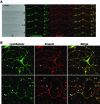
Similar articles
-
AP-3-dependent mechanisms control the targeting of a chloride channel (ClC-3) in neuronal and non-neuronal cells.J Biol Chem. 2004 Jun 11;279(24):25430-9. doi: 10.1074/jbc.M402331200. Epub 2004 Apr 8. J Biol Chem. 2004. PMID: 15073168
-
Dependence of the histofluorescently reactive zinc pool on zinc transporter-3 in the normal brain.Brain Res. 2011 Oct 18;1418:12-22. doi: 10.1016/j.brainres.2011.08.055. Epub 2011 Aug 27. Brain Res. 2011. PMID: 21911210
-
Hermansky-Pudlak protein complexes, AP-3 and BLOC-1, differentially regulate presynaptic composition in the striatum and hippocampus.J Neurosci. 2010 Jan 20;30(3):820-31. doi: 10.1523/JNEUROSCI.3400-09.2010. J Neurosci. 2010. PMID: 20089890 Free PMC article.
-
What is the function of neuronal AP-3?Biol Cell. 2007 Jul;99(7):349-61. doi: 10.1042/BC20070029. Biol Cell. 2007. PMID: 17567262 Review.
-
Neuronal and non-neuronal functions of the AP-3 sorting machinery.J Cell Sci. 2007 Feb 15;120(Pt 4):531-41. doi: 10.1242/jcs.03365. J Cell Sci. 2007. PMID: 17287392 Review.
Cited by
-
Transmembrane 163 (TMEM163) protein interacts with specific mammalian SLC30 zinc efflux transporter family members.Biochem Biophys Rep. 2022 Oct 1;32:101362. doi: 10.1016/j.bbrep.2022.101362. eCollection 2022 Dec. Biochem Biophys Rep. 2022. PMID: 36204728 Free PMC article.
-
Cell-type-specific enhancement of deviance detection by synaptic zinc in the mouse auditory cortex.Proc Natl Acad Sci U S A. 2024 Oct;121(40):e2405615121. doi: 10.1073/pnas.2405615121. Epub 2024 Sep 23. Proc Natl Acad Sci U S A. 2024. PMID: 39312661
-
Two Clathrin Adaptor Protein Complexes Instruct Axon-Dendrite Polarity.Neuron. 2016 May 4;90(3):564-80. doi: 10.1016/j.neuron.2016.04.020. Neuron. 2016. PMID: 27151641 Free PMC article.
-
BLOC-1 complex deficiency alters the targeting of adaptor protein complex-3 cargoes.Mol Biol Cell. 2006 Sep;17(9):4014-26. doi: 10.1091/mbc.e06-02-0103. Epub 2006 Jun 7. Mol Biol Cell. 2006. PMID: 16760431 Free PMC article.
-
Adaptor protein-3 produces synaptic vesicles that release phasic dopamine.Proc Natl Acad Sci U S A. 2023 Oct 17;120(42):e2309843120. doi: 10.1073/pnas.2309843120. Epub 2023 Oct 9. Proc Natl Acad Sci U S A. 2023. PMID: 37812725 Free PMC article.
References
-
- Atwood, H.L., and Karunanithi, S. (2002). Diversification of synaptic strength: presynaptic elements. Nat. Rev. Neurosci. 3, 497-516. - PubMed
-
- Bellocchio, E.E., Reimer, R.J., Fremeau, R.T., Jr., and Edwards, R.H. (2000). Uptake of glutamate into synaptic vesicles by an inorganic phosphate transporter. Science 289, 957-960. - PubMed
Publication types
MeSH terms
Substances
Grants and funding
LinkOut - more resources
Full Text Sources
Research Materials
Miscellaneous