The cell wall of lactic acid bacteria: surface constituents and macromolecular conformations
- PMID: 14645095
- PMCID: PMC1303707
- DOI: 10.1016/S0006-3495(03)74820-6
The cell wall of lactic acid bacteria: surface constituents and macromolecular conformations
Abstract
A variety of strains of the genus Lactobacillus was investigated with respect to the structure, softness, and interactions of their outer surface layers in order to construct structure-property relations of the Gram-positive bacterial cell wall. The role of the conformational properties of the constituents of the outer cell-wall layers and their spatial distribution on the cell wall is emphasized. Atomic force microscopy was used to resolve the surface structure, interactions, and softness of the bacterial cell wall at nanometer-length scales and upwards. The pH-dependence of the electrophoretic mobility and a novel interfacial adhesion assay were used to analyze the average physicochemical properties of the bacterial strains. The bacterial surface is smooth when a compact layer of globular proteins constitutes the outer surface, e.g., the S-layer of L. crispatus DSM20584. In contrast, for two other S-layer containing strains (L. helveticus ATCC12046 and L. helveticus ATCC15009), the S-layer is covered by polymeric surface constituents which adopt a much more extended conformation and which confer a certain roughness to the surface. Consequently, the S-layer is important for the overall surface properties of L. crispatus, but not for the surface properties of L. helveticus. Both surface proteins (L. crispatus DSM20584) and (lipo)teichoic acids (L. johnsonii ATCC332) confer hydrophobic properties to the bacterial surface whereas polysaccharides (L. johnsonii DSM20533 and L. johnsonii ATCC 33200) render the bacterial surface hydrophilic. Using the interfacial adhesion assay, it was demonstrated that hydrophobic groups within the cell wall adsorb limited quantities of hydrophobic compounds. The present work demonstrates that the impressive variation in surface properties displayed by even a limited number of genetically-related bacterial strains can be understood in terms of established colloidal concepts, provided that sufficiently detailed structural, chemical, and conformational information on the surface constituents is available.
Figures
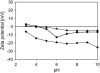
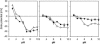
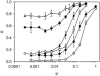
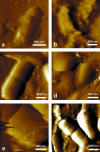
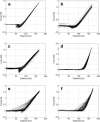
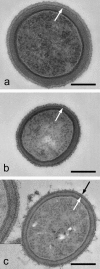
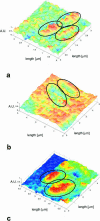
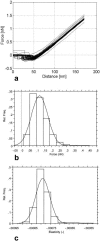
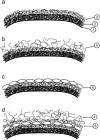
Similar articles
-
Imaging of lactic acid bacteria with AFM--elasticity and adhesion maps and their relationship to biological and structural data.Ultramicroscopy. 2003 Oct-Nov;97(1-4):199-208. doi: 10.1016/S0304-3991(03)00044-5. Ultramicroscopy. 2003. PMID: 12801672
-
Structural studies of the cell wall polysaccharides from three strains of Lactobacillus helveticus with different autolytic properties: DPC4571, BROI, and LH1.Carbohydr Res. 2013 Sep 20;379:7-12. doi: 10.1016/j.carres.2013.05.020. Epub 2013 Jun 12. Carbohydr Res. 2013. PMID: 23831635
-
Fluorescence and atomic force microscopy imaging of wall teichoic acids in Lactobacillus plantarum.ACS Chem Biol. 2011 Apr 15;6(4):366-76. doi: 10.1021/cb1003509. Epub 2011 Feb 4. ACS Chem Biol. 2011. PMID: 21218855
-
Probing bacterial interactions: integrated approaches combining atomic force microscopy, electron microscopy and biophysical techniques.Micron. 2005;36(4):293-320. doi: 10.1016/j.micron.2004.11.005. Epub 2005 Feb 17. Micron. 2005. PMID: 15857770 Review.
-
The biosynthesis and functionality of the cell-wall of lactic acid bacteria.Antonie Van Leeuwenhoek. 1999 Jul-Nov;76(1-4):159-84. Antonie Van Leeuwenhoek. 1999. PMID: 10532377 Review.
Cited by
-
Safety and Beneficial Properties of Bacteriocinogenic Lactococcus lactis and Pediococcus pentosaceus Strains, and Their Effect Versus Oral Cavity Related and Antibiotic-Resistant Pathogens.Probiotics Antimicrob Proteins. 2024 Apr 2. doi: 10.1007/s12602-024-10245-z. Online ahead of print. Probiotics Antimicrob Proteins. 2024. PMID: 38564170
-
Modulation of Zebrafish (Danio rerio) Intestinal Mucosal Barrier Function Fed Different Postbiotics and a Probiotic from Lactobacilli.Microorganisms. 2023 Nov 30;11(12):2900. doi: 10.3390/microorganisms11122900. Microorganisms. 2023. PMID: 38138044 Free PMC article.
-
Electrospinning Microencapsulation of Lactobacillus fermentum K73 Using Gelatin as the Main Component of a Food-Grade Matrix.Microorganisms. 2023 Nov 1;11(11):2682. doi: 10.3390/microorganisms11112682. Microorganisms. 2023. PMID: 38004694 Free PMC article.
-
Promising application of probiotic microorganisms as Pickering emulsions stabilizers.Sci Rep. 2023 Sep 23;13(1):15915. doi: 10.1038/s41598-023-43087-w. Sci Rep. 2023. PMID: 37741896 Free PMC article.
-
Bacteriocin and Antioxidant Production, a Beneficial Properties of Lactic Acid Bacteria Isolated from Fermented Vegetables of Northwest Bulgaria.Probiotics Antimicrob Proteins. 2023 Aug 17. doi: 10.1007/s12602-023-10140-z. Online ahead of print. Probiotics Antimicrob Proteins. 2023. PMID: 37589786
References
-
- Albertsson, P. A. 1986. Partitioning of Cell Particles and Macromolecules, 3rd Ed. Wiley, New York.
-
- Amiel, C., L. Mariey, M.-C. Curk-Daubié, P. Pichon, and J. Travert. 2000. Potentiality of Fourier transform infrared spectroscopy (FTIR) for discrimination and identification of dairy lactic acid bacteria. Lait. 80:445–459.
-
- Atkins, P. W. 1982. Physical Chemistry, 2nd Ed. Oxford University Press, Oxford.
MeSH terms
Substances
LinkOut - more resources
Full Text Sources
Other Literature Sources