Cytokinin-deficient transgenic Arabidopsis plants show multiple developmental alterations indicating opposite functions of cytokinins in the regulation of shoot and root meristem activity
- PMID: 14555694
- PMCID: PMC280559
- DOI: 10.1105/tpc.014928
Cytokinin-deficient transgenic Arabidopsis plants show multiple developmental alterations indicating opposite functions of cytokinins in the regulation of shoot and root meristem activity
Abstract
Cytokinins are hormones that regulate cell division and development. As a result of a lack of specific mutants and biochemical tools, it has not been possible to study the consequences of cytokinin deficiency. Cytokinin-deficient plants are expected to yield information about processes in which cytokinins are limiting and that, therefore, they might regulate. We have engineered transgenic Arabidopsis plants that overexpress individually six different members of the cytokinin oxidase/dehydrogenase (AtCKX) gene family and have undertaken a detailed phenotypic analysis. Transgenic plants had increased cytokinin breakdown (30 to 45% of wild-type cytokinin content) and reduced expression of the cytokinin reporter gene ARR5:GUS (beta-glucuronidase). Cytokinin deficiency resulted in diminished activity of the vegetative and floral shoot apical meristems and leaf primordia, indicating an absolute requirement for the hormone. By contrast, cytokinins are negative regulators of root growth and lateral root formation. We show that the increased growth of the primary root is linked to an enhanced meristematic cell number, suggesting that cytokinins control the exit of cells from the root meristem. Different AtCKX-green fluorescent protein fusion proteins were localized to the vacuoles or the endoplasmic reticulum and possibly to the extracellular space, indicating that subcellular compartmentation plays an important role in cytokinin biology. Analyses of promoter:GUS fusion genes showed differential expression of AtCKX genes during plant development, the activity being confined predominantly to zones of active growth. Our results are consistent with the hypothesis that cytokinins have central, but opposite, regulatory functions in root and shoot meristems and indicate that a fine-tuned control of catabolism plays an important role in ensuring the proper regulation of cytokinin functions.
Figures
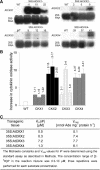
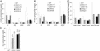
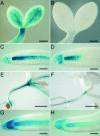
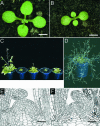
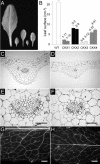
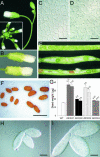
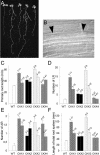
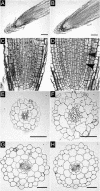
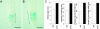
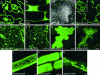
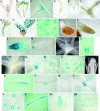
Similar articles
-
Enhanced cytokinin degradation in leaf primordia of transgenic Arabidopsis plants reduces leaf size and shoot organ primordia formation.J Plant Physiol. 2011 Aug 15;168(12):1328-34. doi: 10.1016/j.jplph.2011.03.003. Epub 2011 Apr 6. J Plant Physiol. 2011. PMID: 21474200
-
Overexpression of the cytosolic cytokinin oxidase/dehydrogenase (CKX7) from Arabidopsis causes specific changes in root growth and xylem differentiation.Plant J. 2014 May;78(3):359-71. doi: 10.1111/tpj.12477. Epub 2014 Apr 7. Plant J. 2014. PMID: 24528491
-
Histidine kinase homologs that act as cytokinin receptors possess overlapping functions in the regulation of shoot and root growth in Arabidopsis.Plant Cell. 2004 Jun;16(6):1365-77. doi: 10.1105/tpc.021477. Epub 2004 May 21. Plant Cell. 2004. PMID: 15155880 Free PMC article.
-
WUSCHEL: a master regulator in plant growth signaling.Plant Cell Rep. 2020 Apr;39(4):431-444. doi: 10.1007/s00299-020-02511-5. Epub 2020 Jan 27. Plant Cell Rep. 2020. PMID: 31984435 Review.
-
Grass meristems I: shoot apical meristem maintenance, axillary meristem determinacy and the floral transition.Plant Cell Physiol. 2013 Mar;54(3):302-12. doi: 10.1093/pcp/pct025. Epub 2013 Feb 14. Plant Cell Physiol. 2013. PMID: 23411664 Review.
Cited by
-
Improvement of Rice Agronomic Traits by Editing Type-B Response Regulators.Int J Mol Sci. 2022 Nov 16;23(22):14165. doi: 10.3390/ijms232214165. Int J Mol Sci. 2022. PMID: 36430643 Free PMC article.
-
Cytokinin as a positional cue regulating lateral root spacing in Arabidopsis.J Exp Bot. 2015 Aug;66(15):4759-68. doi: 10.1093/jxb/erv252. Epub 2015 May 27. J Exp Bot. 2015. PMID: 26019251 Free PMC article.
-
OsNAC103, an NAC transcription factor negatively regulates plant height in rice.Planta. 2024 Jan 9;259(2):35. doi: 10.1007/s00425-023-04309-7. Planta. 2024. PMID: 38193994 Free PMC article.
-
Tissue- and Cell-Specific Cytokinin Activity in Populus × canescens Monitored by ARR5::GUS Reporter Lines in Summer and Winter.Front Plant Sci. 2016 May 13;7:652. doi: 10.3389/fpls.2016.00652. eCollection 2016. Front Plant Sci. 2016. PMID: 27242853 Free PMC article.
-
The transcription factor MdBPC2 alters apple growth and promotes dwarfing by regulating auxin biosynthesis.Plant Cell. 2024 Feb 26;36(3):585-604. doi: 10.1093/plcell/koad297. Plant Cell. 2024. PMID: 38019898 Free PMC article.
References
-
- Aloni, R. (1995). The induction of vascular tissues by auxin and cytokinin. In Plant Hormones: Physiology, Biochemistry and Molecular Biology, P.J. Davies, ed (Dordrecht, The Netherlands: Kluwer Academic Publishers), pp. 531–546.
-
- Armstrong, D.J. (1994). Cytokinin oxidase and the regulation of cytokinin degradation. In Cytokinins: Chemistry, Activity, and Function, D.W.S. Mok and M.C. Mok, eds (Boca Raton, FL: CRC Press), pp. 139–154.
-
- Bannai, H., Tamada, Y., Maruyama, O., Nakai, K., and Miyano, S. (2002). Extensive feature detection of N-terminal protein sorting signals. Bioinformatics 18, 298–305. - PubMed
Publication types
MeSH terms
Substances
LinkOut - more resources
Full Text Sources
Other Literature Sources
Molecular Biology Databases
Research Materials