Selective caveolin-1-dependent endocytosis of glycosphingolipids
- PMID: 12925761
- PMCID: PMC181565
- DOI: 10.1091/mbc.e02-12-0809
Selective caveolin-1-dependent endocytosis of glycosphingolipids
Abstract
We studied the endocytosis of fluorescent glycosphingolipid (GSL) analogs in various cell types using pathway-specific inhibitors and colocalization studies with endocytic markers and DsRed caveolin-1 (cav-1). Based on inhibitor studies, all GSLs tested were internalized predominantly (>80%) by a clathrin-independent, caveolar-related mechanism, regardless of cell type. In addition, fluorescent lactosylceramide (LacCer) colocalized with DsRed-cav-1 in vesicular structures upon endocytosis in rat fibroblasts. The internalization mechanism for GSLs was unaffected by varying the carbohydrate headgroup or sphingosine backbone chain length; however, a fluorescent phosphatidylcholine analog was not internalized via caveolae, suggesting that the GSL ceramide core may be important for caveolar uptake. Internalization of fluorescent LacCer was reduced 80-90% in cell types with low cav-1, but was dramatically stimulated by cav-1 overexpression. However, even in cells with low levels of cav-1, residual LacCer internalization was clathrin independent. In contrast, cholera toxin B subunit (CtxB), which binds endogenous GM1, was internalized via clathrin-independent endocytosis in cells with high cav-1 expression, whereas significant clathrin-dependent uptake occurred in cells with low cav-1. Fluorescent GM1, normally internalized by clathrin-independent endocytosis in HeLa cells with low cav-1, was induced to partially internalize via the clathrin pathway in the presence of CtxB. These results suggest that GSL analogs are selectively internalized via a caveolar-related mechanism in most cell types, whereas CtxB may undergo "pathway switching" when cav-1 levels are low.
Figures
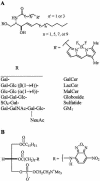
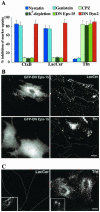
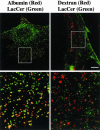
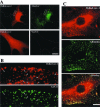
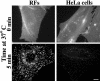
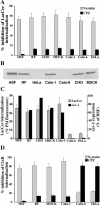
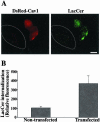
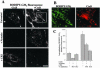
Similar articles
-
Glycosphingolipids internalized via caveolar-related endocytosis rapidly merge with the clathrin pathway in early endosomes and form microdomains for recycling.J Biol Chem. 2003 Feb 28;278(9):7564-72. doi: 10.1074/jbc.M210457200. Epub 2002 Dec 12. J Biol Chem. 2003. PMID: 12482757
-
Selective stimulation of caveolar endocytosis by glycosphingolipids and cholesterol.Mol Biol Cell. 2004 Jul;15(7):3114-22. doi: 10.1091/mbc.e04-03-0189. Epub 2004 Apr 23. Mol Biol Cell. 2004. PMID: 15107466 Free PMC article.
-
Internalization of cholera toxin by different endocytic mechanisms.J Cell Sci. 2001 Oct;114(Pt 20):3737-47. doi: 10.1242/jcs.114.20.3737. J Cell Sci. 2001. PMID: 11707525
-
Caveosomes and endocytosis of lipid rafts.J Cell Sci. 2003 Dec 1;116(Pt 23):4707-14. doi: 10.1242/jcs.00840. J Cell Sci. 2003. PMID: 14600257 Review.
-
Caveolae/raft-dependent endocytosis.J Cell Biol. 2003 May 26;161(4):673-7. doi: 10.1083/jcb.200302028. J Cell Biol. 2003. PMID: 12771123 Free PMC article. Review.
Cited by
-
Fluorescently labelled glycans and their applications.Glycoconj J. 2015 Nov;32(8):559-74. doi: 10.1007/s10719-015-9611-9. Epub 2015 Aug 4. Glycoconj J. 2015. PMID: 26239924 Review.
-
Elevated endosomal cholesterol levels in Niemann-Pick cells inhibit rab4 and perturb membrane recycling.Mol Biol Cell. 2004 Oct;15(10):4500-11. doi: 10.1091/mbc.e04-05-0432. Epub 2004 Aug 3. Mol Biol Cell. 2004. PMID: 15292453 Free PMC article.
-
Ubiquitin C-terminal Hydrolase L1 Regulates Lipid Raft-dependent Endocytosis.Exp Neurobiol. 2018 Oct;27(5):377-386. doi: 10.5607/en.2018.27.5.377. Epub 2018 Oct 31. Exp Neurobiol. 2018. PMID: 30429647 Free PMC article.
-
Synthesis and properties of a photoactivatable analogue of psychosine (beta-Galactosylsphingosine).ChemMedChem. 2010 May 3;5(5):682-6. doi: 10.1002/cmdc.201000018. ChemMedChem. 2010. PMID: 20209561 Free PMC article. No abstract available.
-
Lactosylceramide-enriched microdomains mediate human neutrophil immunological functions via carbohydrate-carbohydrate interaction.Glycoconj J. 2022 Apr;39(2):239-246. doi: 10.1007/s10719-022-10060-0. Epub 2022 Apr 4. Glycoconj J. 2022. PMID: 35377103 Review.
References
-
- Aktories, K., Schmidt, G., and Just, I. (2000). Rho GTPases as targets of bacterial protein toxins. Biol. Chem. 381, 421–426. - PubMed
-
- Anderson, R.G. (1998). The caveolae membrane system. Annu. Rev. Biochem. 67, 199–225. - PubMed
-
- Anderson, R.G., and Jacobson, K. (2002). A role for lipid shells in targeting proteins to caveolae, rafts, and other lipid domains. Science 296, 1821–1825. - PubMed
-
- Aoki, T., Nomura, R., and Fujimoto, T. (1999). Tyrosine phosphorylation of Caveolin-1 in the endothelium. Exp. Cell Res. 253, 629–636. - PubMed
-
- Brown, D.A., and London, E. (2000). Structure and function of sphingolipid- and cholesterol-rich membrane rafts. J. Biol. Chem. 275, 17221–17224. - PubMed
Publication types
MeSH terms
Substances
Grants and funding
LinkOut - more resources
Full Text Sources
Other Literature Sources