Mrc1 is a replication fork component whose phosphorylation in response to DNA replication stress activates Rad53
- PMID: 12865299
- PMCID: PMC196183
- DOI: 10.1101/gad.1098303
Mrc1 is a replication fork component whose phosphorylation in response to DNA replication stress activates Rad53
Abstract
When DNA replication is stalled, a signal transduction pathway is activated that promotes the stability of stalled forks and resumption of DNA synthesis. In budding yeast, this pathway includes the kinases Mec1 and Rad53. Here we report that the Mediator protein Mrc1, which is required for normal DNA replication and for activation of Rad53, is present at replication forks. Mrc1 initially binds early-replicating sequences and moves along chromatin with the replication fork. Blocking initiation of DNA replication blocks Mrc1 loading onto origins, providing an explanation for why so many mutants in DNA replication show checkpoint defects. In the presence of replication blocks, we find that Mec1 is recruited to regions of stalled replication, where it encounters and presumably phosphorylates Mrc1. Mutation of the canonical Mec1 phosphorylation sites on Mrc1 prevents Mrc1 phosphorylation and blocks Rad53 activation, but does not alter Mrc1's role in DNA replication. Our results suggest a model whereby in response to DNA replication interference, the Mec1 kinase is recruited to sites of replication blocks and phosphorylates a component of the DNA replication complex, Mrc1, thereby setting up a solid-state Rad53 activation platform to initiate the checkpoint response.
Figures
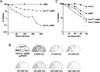
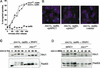
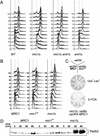
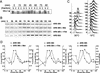
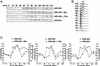
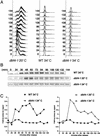
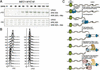
Similar articles
-
Mrc1 phosphorylation in response to DNA replication stress is required for Mec1 accumulation at the stalled fork.Proc Natl Acad Sci U S A. 2009 Aug 4;106(31):12765-70. doi: 10.1073/pnas.0904623106. Epub 2009 Jun 10. Proc Natl Acad Sci U S A. 2009. PMID: 19515819 Free PMC article.
-
Reconstitution of Rad53 activation by Mec1 through adaptor protein Mrc1.J Biol Chem. 2009 Jul 10;284(28):18593-604. doi: 10.1074/jbc.M109.018242. Epub 2009 May 19. J Biol Chem. 2009. PMID: 19457865 Free PMC article.
-
Colocalization of Mec1 and Mrc1 is sufficient for Rad53 phosphorylation in vivo.Mol Biol Cell. 2012 Mar;23(6):1058-67. doi: 10.1091/mbc.E11-10-0852. Epub 2012 Feb 1. Mol Biol Cell. 2012. PMID: 22298423 Free PMC article.
-
Signaling pathways of replication stress in yeast.FEMS Yeast Res. 2017 Mar 1;17(2). doi: 10.1093/femsyr/fow101. FEMS Yeast Res. 2017. PMID: 27915243 Review.
-
The S-phase checkpoint: targeting the replication fork.Biol Cell. 2009 Aug 19;101(11):617-27. doi: 10.1042/BC20090053. Biol Cell. 2009. PMID: 19686094 Review.
Cited by
-
Cohesin association to replication sites depends on rad50 and promotes fork restart.Mol Cell. 2012 Oct 12;48(1):98-108. doi: 10.1016/j.molcel.2012.07.004. Epub 2012 Aug 9. Mol Cell. 2012. PMID: 22885006 Free PMC article.
-
The DNA damage checkpoint: A tale from budding yeast.Front Genet. 2022 Sep 15;13:995163. doi: 10.3389/fgene.2022.995163. eCollection 2022. Front Genet. 2022. PMID: 36186482 Free PMC article. Review.
-
Binding of Multiple Rap1 Proteins Stimulates Chromosome Breakage Induction during DNA Replication.PLoS Genet. 2015 Aug 11;11(8):e1005283. doi: 10.1371/journal.pgen.1005283. eCollection 2015 Aug. PLoS Genet. 2015. PMID: 26263073 Free PMC article.
-
Homologous Recombination: To Fork and Beyond.Genes (Basel). 2018 Dec 4;9(12):603. doi: 10.3390/genes9120603. Genes (Basel). 2018. PMID: 30518053 Free PMC article. Review.
-
Multiple signaling kinases target Mrc1 to prevent genomic instability triggered by transcription-replication conflicts.Nat Commun. 2018 Jan 25;9(1):379. doi: 10.1038/s41467-017-02756-x. Nat Commun. 2018. PMID: 29371596 Free PMC article.
References
-
- Alcasabas A.A., Osborn, A.J., Bachant, J., Hu, F., Werler, P.J., Bousset, K., Furuya, K., Diffley, J.F., Carr, A.M., and Elledge, S.J. 2001. Mrc1 transduces signals of DNA replication stress to activate Rad53. Nat. Cell Biol. 3: 958–965. - PubMed
-
- Allen J.B., Zhou, Z., Siede, W., Friedberg, E.C., and Elledge, S.J. 1994. The SAD1/RAD53 protein kinase controls multiple checkpoints and DNA damage-induced transcription in yeast. Genes & Dev. 8: 2401–2415. - PubMed
-
- Aparicio O.M., Weinstein, D.M., and Bell, S.P. 1997. Components and dynamics of DNA replication complexes in S. cerevisiae: Redistribution of MCM proteins and Cdc45p during S phase. Cell 91: 59–69. - PubMed
Publication types
MeSH terms
Substances
Grants and funding
LinkOut - more resources
Full Text Sources
Other Literature Sources
Molecular Biology Databases
Research Materials
Miscellaneous